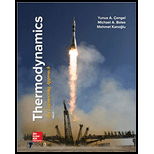
Concept explainers
The process that causes the greatest amount of exergy destruction.

Answer to Problem 117RP
The process that causes the greatest amount of exergy destruction is
Explanation of Solution
Show the T-s diagram for refrigeration system as in Figure (1).
From Figure (1), write the specific enthalpy at state 3 is equal to state 4 due to throttling process.
Here, specific enthalpy at state 3 and 4 is
Express specific enthalpy at state 2 using compressor efficiency.
Here, specific enthalpy at state 2, 1 and 2s is
Express temperature at state 3.
Here, saturation temperature at pressure of
Express quality at state 4.
Here, specific enthalpy at saturation liquid and temperature of
Express specific entropy at state 4.
Here, specific entropy at saturation liquid and temperature of
Express heat rejected in the evaporator.
Here, specific enthalpy at state 4 is
Express heat added in the condenser.
Express the exergy destruction during process 1-2.
Here, surrounding temperature is
Express the exergy destruction during process 2-3.
Here, specific entropy at state 3 is
Express the exergy destruction during process 3-4.
Here, specific entropy at state 4 is
Express the exergy destruction during process 4-1.
Here, freezing temperature of water is
Conclusion:
Refer Table A-11, “saturated refrigerant-134a-temperature table”, and write the properties corresponding to initial temperature
Here, specific entropy at state 1 is
Perform unit conversion of pressure at state 2 from
Refer Table A-13, “superheated refrigerant 134a”, and write the specific enthalpy at state 2s corresponding to pressure at state 2 of
Write the formula of interpolation method of two variables.
Here, the variables denote by x and y is specific entropy at state 2 and specific enthalpy at state 2s respectively.
Show the specific enthalpy at state 2s corresponding to specific entropy as in Table (1).
Specific entropy at state 2 |
Specific enthalpy at state 2s |
0.9314 | 268.47 |
0.9378 | |
0.9642 | 278.59 |
Substitute
Thus, the specific enthalpy at state 2s is,
Refer Table A-12, “saturated refrigerant-134a-pressure table”, and write the saturated temperature corresponding to pressure of
Substitute
Refer Table A-11, “saturated refrigerant-134a-temperature table”, and write the specific enthalpy and entropy at state 3 corresponding to temperature at state 3
Here, specific enthalpy and entropy at saturated liquid is
Substitute
Substitute
Refer Table A-11, “saturated refrigerant-134a-temperature table”, and write the properties corresponding to final temperature
Substitute
Substitute
Refer Table A-13, “superheated refrigerant 134a”, and write the specific entropy at state 2 corresponding to pressure at state 2 of
Show the specific entropy at state 2 corresponding to specific enthalpy as in Table (2).
Specific enthalpy at state 1 |
Specific entropy at state 2 |
268.47 | 0.9314 |
275.02 | |
278.59 | 0.9642 |
Use excels and tabulates the values from Table (2) in Equation (XII) to get,
Substitute
Substitute
Perform unit conversion of temperature from
Substitute
Substitute
Substitute
Take the freezing temperature of water as,
Substitute
Hence, the process that causes the greatest amount of exergy destruction is
Want to see more full solutions like this?
Chapter 11 Solutions
THERMODYNAMICS (LL)-W/ACCESS >CUSTOM<
- TNB steam power plant in Genting Highlandsoperates on reheat Rankine cycleand has power net output of 50MW. Steam enters the turbine at 12MPa and 500 °C and it is cooled in the condenser at a pressure of 12kPa by running cooling water from the Bertam River through the tubesof condenser. Steam enters both stages of the turbine at b°C. If the moisture content of the steam at the exit of the low-pressure turbine is not to exceed 10 percent, show the cycle on a T-s diagram and determine;i.The pressure at which reheating takes place, ii.The thermal efficiency of the cycleandiii.Please justify how does the thermal efficiency of the cycle if the temperature to low turbine is increase to 600°C?arrow_forwardاريد حل كتابة يدarrow_forwardاريد حل كتابة يدarrow_forward
- TNB steam power plant in Genting Highlandsoperates on reheat Rankine cycleand has power net output of 50MW. Steam enters the turbine at aMPa and b °Cand it is cooled in the condenser at a pressure of ckPa by running cooling water from the Bertam River through the tubesof condenser. Steam enters both stages of the turbine at b°C. If the moisture content of the steam at the exit of the low-pressure turbine is not to exceed 10 percent, show the cycle on a T-s diagram and determine;i.The pressure at which reheating takes place, ii.The thermal efficiency of the cycleandiii.Please justify how does the thermal efficiency of the cycle if the temperature to low turbine is increase to 600°C? a=12 b=500 c=12arrow_forwardThe evaporator and condenser temperatures are -20°C and 35°C, respectively, in an ammonia simple saturation cycle. The method will be used to produce 12,500kg of ice at -15°C in 32 hours from water at 25°C. Assuming that 25 percent of the heat absorbed from the water is lost. Ice has a specific heat of 2.094Kj/kg-K, while fusion has a heat of 335kj/kg. Determine the: a) Total Heat b) Mass flow ratearrow_forwardIn a gas turbine generating set two stages of compression are used with an intercooler between stages. The HP turbine drives the HP compressor, and the LP turbine drives the LP compressor and the generator. The exhaust from the LP turbine through a heat exchanger which transfer heat to the air leaving the HP compressor. There is a reheat combustion chamber between turbine stages which raises the gas temperature to 8000C, which is also the gas temperature at entry to the HP turbine. The overall pressure ratio is 30, each compressor having the same pressure ratio, and the air temperature at entry to the unit is 300C. The heat exchanger thermal ratio may be taken as 0.75, and intercooling is complete between compressor stages. Assume isentropic efficiencies of 0.85 for both compressor stages, and 0.9 for both turbine stages, and the 4% of the work of each turbine is used in overcoming friction. Neglecting the losses in pressure, and assuming that velocity changes are negligibly small,…arrow_forward
- An ideal vapor-compression refrigeration cycle using refrigerant-134a as the working fluid is used to cool a brine solution to -5°C. This solution is pumped to various buildings for the purpose of air-conditioning. The refrigerant evaporates at -12.00°C with a total mass flow rate of 8.000 kg/s and condenses at 600 kPa. Determine the COP of the cycle and the total cooling load. (Take the required values from saturated refrigerant-134a tables.) The COP of the cycle is (Round the final answer to three decimal places.) and the total cooling load is kW.arrow_forwardThermodynamicarrow_forwardThe performance of a refrigeration system is measured and found to have a COP of 2.5. The refrigerant is ammonia (R717). The saturated evaporation temperature is -10.0°C, and the saturated condensation temperature is 45.0°C. The liquid leaving the condenser is subcooled by 7.5°C. There is no superheating. Estimate the apparent isentropic efficiency of the compressor. Give your answer to 2DP.arrow_forward
- Draw the T-S diagramNotes: Assume the steam is reheated to the inlet temperature of the high-pressure turbine. The moisture content of the steam at the exit of the low-pressure turbine is not to exceed 9.5%arrow_forwardIn a steam turbine plant steam expands in the high pressure turbine from 5000kPa, 500°C to 500kPa with an isentropic efficiency of 0.9. It is then reheated isobarically to 500°C before entering the low pressure turbine. In the low pressure turbine the steam expands to dry saturated, 5kPa. The condensate leaves the condenser with 4° of undercooling. Determine: (a). the condition of the high presfsure turbine exhaust (b). the isentropic efficiency of the low pressure turbine (c). the specific steam consumption for a turbine output power of 7.5MW (d). the Rankine efficiency including the feed pumparrow_forwardI need the answer as soon as possiblearrow_forward
- Elements Of ElectromagneticsMechanical EngineeringISBN:9780190698614Author:Sadiku, Matthew N. O.Publisher:Oxford University PressMechanics of Materials (10th Edition)Mechanical EngineeringISBN:9780134319650Author:Russell C. HibbelerPublisher:PEARSONThermodynamics: An Engineering ApproachMechanical EngineeringISBN:9781259822674Author:Yunus A. Cengel Dr., Michael A. BolesPublisher:McGraw-Hill Education
- Control Systems EngineeringMechanical EngineeringISBN:9781118170519Author:Norman S. NisePublisher:WILEYMechanics of Materials (MindTap Course List)Mechanical EngineeringISBN:9781337093347Author:Barry J. Goodno, James M. GerePublisher:Cengage LearningEngineering Mechanics: StaticsMechanical EngineeringISBN:9781118807330Author:James L. Meriam, L. G. Kraige, J. N. BoltonPublisher:WILEY
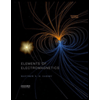
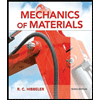
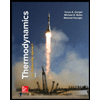
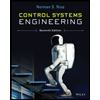
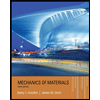
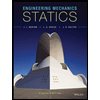