replaced by black pigments. These results show that optix is re- quired for red color pattern specification in H. erato, and acts as a coordinating "or" switch between ommochrome (orange and red) and melanin (black and gray) pigment types. To test whether optix has a role in color patterning in a more basal heliconiine butterfly, we generated knockouts in the gulf fritillary A. vanillae (Fig. 1 D-F, Fig. S1, and Dataset S1, Tables S1 and S2). Previous in situ hybridization work in A. vanillae suggested that optix is not expressed in association with ommo- chrome patterns during early pupal development, leading to the hypothesis that the gene might not play a major color patterning role in this species (14). Thus, we were surprised to find that optix knockout resulted in a complete transformation of ommo- chrome scales to black melanin scales, producing a very unusual and dramatic phenotype of a completely black and silver butterfly (Fig. 1D). We also observed a handful of orange or brown scales that changed to silver patches (Fig. 1D, ventral forewing, green arrows), although we cannot confidently conclude that these are cell- autonomous knockout effects since it has been shown that silver scales can be induced through long-range signaling (18, 19), in this case potentially from neighboring knockout clones. The wild-type (WT) black spots and marginal bands in the ventral forewing were unaffected in knockouts and remained a darker color relative to the neighboring mutant melanic scales (Fig. 1F). optix knockout also resulted in melanic hyperpigmentation in adult bodies (Fig. S1A). Thus, our results in A. vanillae are consistent with those in H. erato in supporting a role for optix as a switch-like regulator that toggles between ommochrome and melanin patterns. We next aimed to test whether optix regulates wing patterning in more distantly related lineages by performing knockouts in the nymphalines V. cardui (Fig. 2, Fig. S2, and Dataset S1, Tables S1 and S2) and J. coenia (Fig. 3, Fig. S3, and Dataset S1, Tables S1 and S2), which diverged from heliconiines by ~75-80 mya (20, 21). Our results were consistent with those from H. erato and A. vanillae, where optix knockouts in both species showed mutant clones with complete loss of presumptive ommochrome pig- ments and replacement by melanins (Figs. 2 A-E and 3 A-C). One interesting exception to this finding was in V. cardui, where 10708 | www.pnas.org/cgi/doi/10.1073/pnas.1709058114 C and G, 2F, and 3F). Furthermore, in H. erato, A. vanillae, and V. cardui, where wing conjugation scales display color pigmentation, we observed both structural and pigmentation changes in the same scales, suggesting that optix can coregulate both scale morphology and pigmentation simultaneously. One final observation of note relates to the optix-expressing pheroscales that occur along the veins of male A. vanillae (14). These scales did not show any grossly ap- parent transformation in optix knockouts (data not shown), even though the scales occurred within obvious knockout clones. Therefore, whether optix plays a functional role in the development of pheroscales, as was predicted previously (14), remains an open question. In sum, our observations that optix knockout results in transformation of wing conjugation scales to normal wing scales V. V. cordu Fig. 2. optix determines wing scale color identity and morphology in V. cardui. (A) optix knockout mutant showing loss of ommochrome pigments. (B-D) Left-right asymmetrical comparisons from individual optix mutant but terflies, showing melanization of red patterns (8), loss of color pigmentation without widespread hypermelanization in the ventral forewing (C) and hindwing (D). (E) Severe defects in late stage pupal wings displaying hypermelanization in red regions of dorsal and ventral wing surfaces (green and purple arrowheads) compared with mosaic adult mutants in A. (F) optix knockout showing con version of pointed wing conjugation scales to normal scales. Zhang et al. Fig. 1. optix determines wing scale color identity and morphology in H. erato and A. vanillae. (A) optix mosaic knockouts in H. erato result in con- version of red ommochrome color patterns to black melanin. The compari sons shown are left-right asymmetrical knockout effects from single individual injected butterflies. (B) Detail of mutant clone highlighted in the mutant in A showing red replaced by black in a proximal red "dennis" pattern of the dorsal forewing. (C-C") optix knockout mosaics showing transformation of pointed wing conjugation scales to normal wing scales. Each panel in the series shows successive detail. (D) optix replaces orange and brown ommochromes in A. vanillae with melanins, resulting in a black and silver butterfly. Arrows highlight presumptive done boundaries dis cussed in the text. (E) Detail of a knockout clone boundary highlighting the switch between red and black pigmentation in the ventral forewing from D. (F) Ventral view of black spots in optix knockout mutant showing a phe- notype similar to WT. (G and G) Wing conjugation scales in WT (G) and optix knockout mutant (G) demonstrating a role for optix in determining A. vanillae scale morphology. replaced by black pigments. These results show that optix is re- quired for red color pattern specification in H. erato, and acts as a coordinating "or" switch between ommochrome (orange and red) and melanin (black and gray) pigment types. To test whether optix has a role in color patterning in a more basal heliconiine butterfly, we generated knockouts in the gulf fritillary A. vanillae (Fig. 1 D-F, Fig. S1, and Dataset S1, Tables S1 and S2). Previous in situ hybridization work in A. vanillae suggested that optix is not expressed in association with ommo- chrome patterns during early pupal development, leading to the hypothesis that the gene might not play a major color patterning role in this species (14). Thus, we were surprised to find that optix knockout resulted in a complete transformation of ommo- chrome scales to black melanin scales, producing a very unusual and the complete ommochrome-to-melanin switch consistently oc- curred in dorsal wings (Fig. 2A and B), but much of the ventral wing area showed only a loss of ommochrome and little obvious hypermelanization (Fig. 2 A, C, and D). Importantly, however, we recovered late-stage pupal wings from V. cardui that had died before emergence that displayed hypermelanization of ventral wing surfaces (Fig. 3E). We speculate that this variable strength of ventral wing pattern melanization among individuals may re- flect a dosage effect, with the stronger phenotypes representing biallelic optix deletion clones. We have no direct evidence for this, however, given the challenges in rigorously characterizing specific alleles from individual mutant clones (16). We also re- covered hypermelanic optix knockout pupae in both V. cardui (Fig. S2) and J. coenia (Fig. S3). Taken together, our knockout data from four nymphalids clearly demonstrate that optix plays a conserved role in coordinating the color identities of butterfly wing scales, where it operates as an "or" function between ommochrome and melanin identities, but also may be modulated to serve as an "and" function in some contexts, as demonstrated by phenotypes seen in the ventral wings of V. cardui. optix Function Is Required for Determination of Derived Scale Structures. Along with its expression in color patterns, in situ optix expression also precisely predicts the location of patches of derived, pointed scales thought to play a role in conjugating forewings and hindwings during flight (5, 14). To determine whether optix plays a role in determining the unusual morphology of these scales, we examined optix knockouts for changes in wing scale structure. Indeed, we found that in all four species, optix knockout resulted in trans- formation of wing conjugation scales to normal wing scales (Figs. 1 C and G, 2F, and 3F). Furthermore, in H. erato, A. vanillae, and V. cardui, where wing conjugation scales display color pigmentation, we observed both structural and pigmentation changes in the same scales, suggesting that optix can coregulate both scale morphology and pigmentation simultaneously. One final observation of note relates to the optix-expressing pheroscales that occur along the veins of male A. vanillae (14). These scales did not show any grossly ap- parent transformation in optix knockouts (data not shown), even though the scales occurred within obvious knockout clones. Therefore, whether optix plays a functional role in the development of pheroscales, as was predicted previously (14), remains an open question. In sum, our observations that optix knockout results in transformation of wing conjugation scales to normal wing scales
replaced by black pigments. These results show that optix is re- quired for red color pattern specification in H. erato, and acts as a coordinating "or" switch between ommochrome (orange and red) and melanin (black and gray) pigment types. To test whether optix has a role in color patterning in a more basal heliconiine butterfly, we generated knockouts in the gulf fritillary A. vanillae (Fig. 1 D-F, Fig. S1, and Dataset S1, Tables S1 and S2). Previous in situ hybridization work in A. vanillae suggested that optix is not expressed in association with ommo- chrome patterns during early pupal development, leading to the hypothesis that the gene might not play a major color patterning role in this species (14). Thus, we were surprised to find that optix knockout resulted in a complete transformation of ommo- chrome scales to black melanin scales, producing a very unusual and dramatic phenotype of a completely black and silver butterfly (Fig. 1D). We also observed a handful of orange or brown scales that changed to silver patches (Fig. 1D, ventral forewing, green arrows), although we cannot confidently conclude that these are cell- autonomous knockout effects since it has been shown that silver scales can be induced through long-range signaling (18, 19), in this case potentially from neighboring knockout clones. The wild-type (WT) black spots and marginal bands in the ventral forewing were unaffected in knockouts and remained a darker color relative to the neighboring mutant melanic scales (Fig. 1F). optix knockout also resulted in melanic hyperpigmentation in adult bodies (Fig. S1A). Thus, our results in A. vanillae are consistent with those in H. erato in supporting a role for optix as a switch-like regulator that toggles between ommochrome and melanin patterns. We next aimed to test whether optix regulates wing patterning in more distantly related lineages by performing knockouts in the nymphalines V. cardui (Fig. 2, Fig. S2, and Dataset S1, Tables S1 and S2) and J. coenia (Fig. 3, Fig. S3, and Dataset S1, Tables S1 and S2), which diverged from heliconiines by ~75-80 mya (20, 21). Our results were consistent with those from H. erato and A. vanillae, where optix knockouts in both species showed mutant clones with complete loss of presumptive ommochrome pig- ments and replacement by melanins (Figs. 2 A-E and 3 A-C). One interesting exception to this finding was in V. cardui, where 10708 | www.pnas.org/cgi/doi/10.1073/pnas.1709058114 C and G, 2F, and 3F). Furthermore, in H. erato, A. vanillae, and V. cardui, where wing conjugation scales display color pigmentation, we observed both structural and pigmentation changes in the same scales, suggesting that optix can coregulate both scale morphology and pigmentation simultaneously. One final observation of note relates to the optix-expressing pheroscales that occur along the veins of male A. vanillae (14). These scales did not show any grossly ap- parent transformation in optix knockouts (data not shown), even though the scales occurred within obvious knockout clones. Therefore, whether optix plays a functional role in the development of pheroscales, as was predicted previously (14), remains an open question. In sum, our observations that optix knockout results in transformation of wing conjugation scales to normal wing scales V. V. cordu Fig. 2. optix determines wing scale color identity and morphology in V. cardui. (A) optix knockout mutant showing loss of ommochrome pigments. (B-D) Left-right asymmetrical comparisons from individual optix mutant but terflies, showing melanization of red patterns (8), loss of color pigmentation without widespread hypermelanization in the ventral forewing (C) and hindwing (D). (E) Severe defects in late stage pupal wings displaying hypermelanization in red regions of dorsal and ventral wing surfaces (green and purple arrowheads) compared with mosaic adult mutants in A. (F) optix knockout showing con version of pointed wing conjugation scales to normal scales. Zhang et al. Fig. 1. optix determines wing scale color identity and morphology in H. erato and A. vanillae. (A) optix mosaic knockouts in H. erato result in con- version of red ommochrome color patterns to black melanin. The compari sons shown are left-right asymmetrical knockout effects from single individual injected butterflies. (B) Detail of mutant clone highlighted in the mutant in A showing red replaced by black in a proximal red "dennis" pattern of the dorsal forewing. (C-C") optix knockout mosaics showing transformation of pointed wing conjugation scales to normal wing scales. Each panel in the series shows successive detail. (D) optix replaces orange and brown ommochromes in A. vanillae with melanins, resulting in a black and silver butterfly. Arrows highlight presumptive done boundaries dis cussed in the text. (E) Detail of a knockout clone boundary highlighting the switch between red and black pigmentation in the ventral forewing from D. (F) Ventral view of black spots in optix knockout mutant showing a phe- notype similar to WT. (G and G) Wing conjugation scales in WT (G) and optix knockout mutant (G) demonstrating a role for optix in determining A. vanillae scale morphology. replaced by black pigments. These results show that optix is re- quired for red color pattern specification in H. erato, and acts as a coordinating "or" switch between ommochrome (orange and red) and melanin (black and gray) pigment types. To test whether optix has a role in color patterning in a more basal heliconiine butterfly, we generated knockouts in the gulf fritillary A. vanillae (Fig. 1 D-F, Fig. S1, and Dataset S1, Tables S1 and S2). Previous in situ hybridization work in A. vanillae suggested that optix is not expressed in association with ommo- chrome patterns during early pupal development, leading to the hypothesis that the gene might not play a major color patterning role in this species (14). Thus, we were surprised to find that optix knockout resulted in a complete transformation of ommo- chrome scales to black melanin scales, producing a very unusual and the complete ommochrome-to-melanin switch consistently oc- curred in dorsal wings (Fig. 2A and B), but much of the ventral wing area showed only a loss of ommochrome and little obvious hypermelanization (Fig. 2 A, C, and D). Importantly, however, we recovered late-stage pupal wings from V. cardui that had died before emergence that displayed hypermelanization of ventral wing surfaces (Fig. 3E). We speculate that this variable strength of ventral wing pattern melanization among individuals may re- flect a dosage effect, with the stronger phenotypes representing biallelic optix deletion clones. We have no direct evidence for this, however, given the challenges in rigorously characterizing specific alleles from individual mutant clones (16). We also re- covered hypermelanic optix knockout pupae in both V. cardui (Fig. S2) and J. coenia (Fig. S3). Taken together, our knockout data from four nymphalids clearly demonstrate that optix plays a conserved role in coordinating the color identities of butterfly wing scales, where it operates as an "or" function between ommochrome and melanin identities, but also may be modulated to serve as an "and" function in some contexts, as demonstrated by phenotypes seen in the ventral wings of V. cardui. optix Function Is Required for Determination of Derived Scale Structures. Along with its expression in color patterns, in situ optix expression also precisely predicts the location of patches of derived, pointed scales thought to play a role in conjugating forewings and hindwings during flight (5, 14). To determine whether optix plays a role in determining the unusual morphology of these scales, we examined optix knockouts for changes in wing scale structure. Indeed, we found that in all four species, optix knockout resulted in trans- formation of wing conjugation scales to normal wing scales (Figs. 1 C and G, 2F, and 3F). Furthermore, in H. erato, A. vanillae, and V. cardui, where wing conjugation scales display color pigmentation, we observed both structural and pigmentation changes in the same scales, suggesting that optix can coregulate both scale morphology and pigmentation simultaneously. One final observation of note relates to the optix-expressing pheroscales that occur along the veins of male A. vanillae (14). These scales did not show any grossly ap- parent transformation in optix knockouts (data not shown), even though the scales occurred within obvious knockout clones. Therefore, whether optix plays a functional role in the development of pheroscales, as was predicted previously (14), remains an open question. In sum, our observations that optix knockout results in transformation of wing conjugation scales to normal wing scales
Biology: The Dynamic Science (MindTap Course List)
4th Edition
ISBN:9781305389892
Author:Peter J. Russell, Paul E. Hertz, Beverly McMillan
Publisher:Peter J. Russell, Paul E. Hertz, Beverly McMillan
Chapter15: From Dna To Protein
Section15.1: The Connection Between Dna, Rna, And Protein
Problem 1SB
Related questions
Question
a) What do the authors say is known and not known about the genetics of butterfly wing patterns?
b) What were the controls and experimental manipulations in this experiment? Give specific examples of each from Figure 1.
c) Carefully read the the Figure 1 legend. Annotate the copy of Figure 1 by following the instructions in the boxes below. Write everything in your own words and in a way that a general audience would understand.

Transcribed Image Text:replaced by black pigments. These results show that optix is re-
quired for red color pattern specification in H. erato, and acts as a
coordinating "or" switch between ommochrome (orange and
red) and melanin (black and gray) pigment types.
To test whether optix has a role in color patterning in a more
basal heliconiine butterfly, we generated knockouts in the gulf
fritillary A. vanillae (Fig. 1 D-F, Fig. S1, and Dataset S1, Tables
S1 and S2). Previous in situ hybridization work in A. vanillae
suggested that optix is not expressed in association with ommo-
chrome patterns during early pupal development, leading to the
hypothesis that the gene might not play a major color patterning
role in this species (14). Thus, we were surprised to find that
optix knockout resulted in a complete transformation of ommo-
chrome scales to black melanin scales, producing a very unusual and
dramatic phenotype of a completely black and silver butterfly (Fig.
1D). We also observed a handful of orange or brown scales that
changed to silver patches (Fig. 1D, ventral forewing, green arrows),
although we cannot confidently conclude that these are cell-
autonomous knockout effects since it has been shown that silver
scales can be induced through long-range signaling (18, 19), in this
case potentially from neighboring knockout clones. The wild-type
(WT) black spots and marginal bands in the ventral forewing were
unaffected in knockouts and remained a darker color relative to the
neighboring mutant melanic scales (Fig. 1F). optix knockout also
resulted in melanic hyperpigmentation in adult bodies (Fig. S1A).
Thus, our results in A. vanillae are consistent with those in H. erato
in supporting a role for optix as a switch-like regulator that toggles
between ommochrome and melanin patterns.
We next aimed to test whether optix regulates wing patterning
in more distantly related lineages by performing knockouts in the
nymphalines V. cardui (Fig. 2, Fig. S2, and Dataset S1, Tables
S1 and S2) and J. coenia (Fig. 3, Fig. S3, and Dataset S1, Tables
S1 and S2), which diverged from heliconiines by ~75-80 mya (20,
21). Our results were consistent with those from H. erato and
A. vanillae, where optix knockouts in both species showed mutant
clones with complete loss of presumptive ommochrome pig-
ments and replacement by melanins (Figs. 2 A-E and 3 A-C).
One interesting exception to this finding was in V. cardui, where
10708 | www.pnas.org/cgi/doi/10.1073/pnas.1709058114
C and G, 2F, and 3F). Furthermore, in H. erato, A. vanillae, and
V. cardui, where wing conjugation scales display color pigmentation,
we observed both structural and pigmentation changes in the same
scales, suggesting that optix can coregulate both scale morphology
and pigmentation simultaneously. One final observation of note
relates to the optix-expressing pheroscales that occur along the veins
of male A. vanillae (14). These scales did not show any grossly ap-
parent transformation in optix knockouts (data not shown), even
though the scales occurred within obvious knockout clones.
Therefore, whether optix plays a functional role in the development
of pheroscales, as was predicted previously (14), remains an open
question. In sum, our observations that optix knockout results in
transformation of wing conjugation scales to normal wing scales
V.
V. cordu
Fig. 2. optix determines wing scale color identity and morphology in
V. cardui. (A) optix knockout mutant showing loss of ommochrome pigments.
(B-D) Left-right asymmetrical comparisons from individual optix mutant but
terflies, showing melanization of red patterns (8), loss of color pigmentation
without widespread hypermelanization in the ventral forewing (C) and hindwing
(D). (E) Severe defects in late stage pupal wings displaying hypermelanization in
red regions of dorsal and ventral wing surfaces (green and purple arrowheads)
compared with mosaic adult mutants in A. (F) optix knockout showing con
version of pointed wing conjugation scales to normal scales.
Zhang et al.

Transcribed Image Text:Fig. 1. optix determines wing scale color identity and morphology in H.
erato and A. vanillae. (A) optix mosaic knockouts in H. erato result in con-
version of red ommochrome color patterns to black melanin. The compari
sons shown are left-right asymmetrical knockout effects from single
individual injected butterflies. (B) Detail of mutant clone highlighted in the
mutant in A showing red replaced by black in a proximal red "dennis"
pattern of the dorsal forewing. (C-C") optix knockout mosaics showing
transformation of pointed wing conjugation scales to normal wing scales.
Each panel in the series shows successive detail. (D) optix replaces orange
and brown ommochromes in A. vanillae with melanins, resulting in a black
and silver butterfly. Arrows highlight presumptive done boundaries dis
cussed in the text. (E) Detail of a knockout clone boundary highlighting the
switch between red and black pigmentation in the ventral forewing from D.
(F) Ventral view of black spots in optix knockout mutant showing a phe-
notype similar to WT. (G and G) Wing conjugation scales in WT (G) and optix
knockout mutant (G) demonstrating a role for optix in determining A.
vanillae scale morphology.
replaced by black pigments. These results show that optix is re-
quired for red color pattern specification in H. erato, and acts as a
coordinating "or" switch between ommochrome (orange and
red) and melanin (black and gray) pigment types.
To test whether optix has a role in color patterning in a more
basal heliconiine butterfly, we generated knockouts in the gulf
fritillary A. vanillae (Fig. 1 D-F, Fig. S1, and Dataset S1, Tables
S1 and S2). Previous in situ hybridization work in A. vanillae
suggested that optix is not expressed in association with ommo-
chrome patterns during early pupal development, leading to the
hypothesis that the gene might not play a major color patterning
role in this species (14). Thus, we were surprised to find that
optix knockout resulted in a complete transformation of ommo-
chrome scales to black melanin scales, producing a very unusual and
the complete ommochrome-to-melanin switch consistently oc-
curred in dorsal wings (Fig. 2A and B), but much of the ventral
wing area showed only a loss of ommochrome and little obvious
hypermelanization (Fig. 2 A, C, and D). Importantly, however,
we recovered late-stage pupal wings from V. cardui that had died
before emergence that displayed hypermelanization of ventral
wing surfaces (Fig. 3E). We speculate that this variable strength
of ventral wing pattern melanization among individuals may re-
flect a dosage effect, with the stronger phenotypes representing
biallelic optix deletion clones. We have no direct evidence for
this, however, given the challenges in rigorously characterizing
specific alleles from individual mutant clones (16). We also re-
covered hypermelanic optix knockout pupae in both V. cardui
(Fig. S2) and J. coenia (Fig. S3). Taken together, our knockout
data from four nymphalids clearly demonstrate that optix plays a
conserved role in coordinating the color identities of butterfly
wing scales, where it operates as an "or" function between
ommochrome and melanin identities, but also may be modulated
to serve as an "and" function in some contexts, as demonstrated
by phenotypes seen in the ventral wings of V. cardui.
optix Function Is Required for Determination of Derived Scale Structures.
Along with its expression in color patterns, in situ optix expression
also precisely predicts the location of patches of derived, pointed
scales thought to play a role in conjugating forewings and hindwings
during flight (5, 14). To determine whether optix plays a role in
determining the unusual morphology of these scales, we examined
optix knockouts for changes in wing scale structure. Indeed, we
found that in all four species, optix knockout resulted in trans-
formation of wing conjugation scales to normal wing scales (Figs. 1
C and G, 2F, and 3F). Furthermore, in H. erato, A. vanillae, and
V. cardui, where wing conjugation scales display color pigmentation,
we observed both structural and pigmentation changes in the same
scales, suggesting that optix can coregulate both scale morphology
and pigmentation simultaneously. One final observation of note
relates to the optix-expressing pheroscales that occur along the veins
of male A. vanillae (14). These scales did not show any grossly ap-
parent transformation in optix knockouts (data not shown), even
though the scales occurred within obvious knockout clones.
Therefore, whether optix plays a functional role in the development
of pheroscales, as was predicted previously (14), remains an open
question. In sum, our observations that optix knockout results in
transformation of wing conjugation scales to normal wing scales
AI-Generated Solution
Unlock instant AI solutions
Tap the button
to generate a solution
Recommended textbooks for you
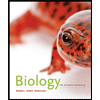
Biology: The Dynamic Science (MindTap Course List)
Biology
ISBN:
9781305389892
Author:
Peter J. Russell, Paul E. Hertz, Beverly McMillan
Publisher:
Cengage Learning
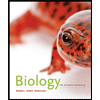
Biology: The Dynamic Science (MindTap Course List)
Biology
ISBN:
9781305389892
Author:
Peter J. Russell, Paul E. Hertz, Beverly McMillan
Publisher:
Cengage Learning