Microtubule motors in mitosis David J. Sharp, Gregory C. Rogers & Jonathan M. Scholey Section of Molecular and Cellular Biology, University of California-Davis, I Shields Ave, Davis, California 95616, USA The mitotic spindle uses microtubule-based motor proteins to assemble itself and to segregate sister chromatids. It is becoming clear that motors invoke several distinct mechanisms to generate the forces that drive mitosis. Moreover, in carrying out its function, the spindle appears to pass through a series of transient steady-state structures, each established by a delicate balance of forces generated by multiple complementary and antagonistic motors. Transitions from one steady state to the next can occur when a change in the activity of a subset of mitotic motors tips the balance. T he mitotic spindle is a bipolar, self-organizing microtubule (MT)-based machine that uses energy liberated from nucleotide hydrolysis to segregate sister chromatids accurately into the daughter cells during cell division¹². In this review we focus on two inter-related aspects of spindle action: (1) spindle morphogenesis, characterized by the separation of the spindle poles that occurs during spindle assembly, maintenance and elongation, and (2) chromosome movements that occur during the capture of chromosomes, their alignment on the spindle equator and the segregation of sister chromatids (Fig. 1). Mitotic-spindle function and design The mechanism of spindle action has long fascinated biologists and during the past 50 years, two critical advances were made. First, the observation that the spindle is a very dynamic structure, superficially resembling a liquid crystal', led to the discovery that spindle MTs dis- play dynamic instability, undergoing transitions between episodes of polymerization and depolymerization that are coupled to GTP hydrolysis'. The dynamic properties of MTs are obviously important for spindle morphogenesis as well as force generation within the spindle. Second, the proposal that mitotic movements could be explained by a 'sliding filament' mechanism' led to the elucidation of the structural organization of spindle MTs (Fig. 2) and a search for mitotic motors that could use ATP hydrolysis to drive the sliding of MTs relative to adjacent MTs or to other spindle structures. These motors form the focus of this review. MT-based motor proteins form two families of ATP-dependent force-generating enzymes, the kinesins and the dyneins". Following the seminal discovery of two kinesin motors with mitotic func- tions it has become established that a strikingly large number of MT-based motors participate in spindle action (Table 1). During the past decade, great strides have been made in our understanding of the basic motor mechanisms of mitosis. Surprisingly, it appears that mitotic motors use at least three distinct mechanisms: (1) cross- bridging and sliding MTs relative to adjacent MTs or other structures; (2) transporting specific mitotic cargoes along the surface lattice of spindle MTs; and (3) regulating MT assembly dynamics and cou- pling movement to MT growth and shrinkage (see Box 1). In addi- tion, analyses of the functional inter-relationships between multiple mitotic motors have revealed that specific mitotic movements are not driven by individual motors but, instead, result from shifts in a dynamic balance of complementary and antagonistic forces generat- ed by multiple motors functioning co-operatively. Mitotic motor mechanisms MT sliding. It has long been proposed that force is generated in the spindle by motor-driven MT sliding". For example, motors could crosslink and slide overlapping antiparallel MTs within interpolar MT bundles to position spindle poles in relation to one another. A growing body of evidence indicates that motors actually use two dis- NATURE VOL 407|7 SEPTEMBER 2000 | www.nature.com KEY Microtubule Chromosome arm Centriole Kinetochore Figure 1 Spindle pole and chromosome movements during mitosis. The activities of MTs and their associated motors are responsible for the carefully orchestrated sequence of movements that underlie mitosis. Early in mitosis (prophase and prometaphase) the bipolar spindle assembles (a) and condensed chromosomes are captured by spindle MTs and congress at the spindle equator (d). By metaphase, the spindle consists of two partially interdigitating arrays of MTs emanating from the duplicated centrosomes that form the two spindle poles (b). Chromosomes lie on the equator with pairs of identical sister chromatids facing opposite spindle poles (e). Chromatid segregation involves both the movement of sister chromatids to opposite spindle poles (t; anaphase A) and the elongation of the spindle itself (c; anaphase B). Mechanistically it is convenient to think of these events in terms of spindle assembly. maintenance and elongation (left), and chromosome capture, congression and segregation (right). MT dynamics and length adjustments are in refs 2,5. 2000 Macmillan Magazines Ltd 41 Mapping trends in insecticide resistance phenotypes in African malaria v... https://journals.plos.org/plosbiology/article?id=10.1371/journal.pbio.30... 1 of 17 Mapping trends in insecticide resistance phenotypes in African malaria vectors Penelope A. Hancock, Chantal J. M. Hendriks, Julie-Anne Tangena, Harry Gibson, Janet Hemingway, Michael Coleman, Peter W. Gething, Ewan Cameron, Samir Bhatt, Catherine L. Moyes Published: June 25, 2020 • https://doi.org/10.1371/journal.pbio.3000633 Abstract Mitigating the threat of insecticide resistance in African malaria vector populations requires comprehensive information about where resistance occurs, to what degree, and how this has changed over time. Estimating these trends is complicated by the sparse, heterogeneous distribution of observations of resistance phenotypes in field populations. We use 6,423 observations of the prevalence of resistance to the most important vector control insecticides to inform a Bayesian geostatistical ensemble modelling approach, generating fine-scale predictive maps of resistance phenotypes in mosquitoes from the Anopheles gambiae complex across Africa. Our models are informed by a suite of 111 predictor variables describing potential drivers of selection for resistance. Our maps show alarming increases in the prevalence of resistance to pyrethroids and DDT across sub-Saharan Africa from 2005 to 2017, with mean mortality following insecticide exposure declining from almost 100% to less than 30% in some areas, as well as substantial spatial variation in resistance trends. Citation: Hancock PA, Hendriks CJM, Tangena J-A, Gibson H, Hemingway J, Coleman M, et al. (2020) Mapping trends in insecticide resistance phenotypes in African malaria vectors. PLoS Biol 18(6):e3000633. https://doi.org/10.1371 /journal.pbio.3000633 Academic Editor: Andrew Fraser Read, The Pennsylvania State University, UNITED STATES Received: December 16, 2019; Accepted: May 11, 2020; Published: June 25, 2020 Copyright: ©2020 Hancock et al. This is an open access article distributed under the terms of the Creative Commons Attribution License, which permits unrestricted use, distribution, and reproduction in any medium, provided the original author and source are credited. Data Availability: The predictive maps of the mean prevalence of resistance are available to download from Figshare (10.6084/m9.figshare.9912623) and will be available to visualise on the Malaria Atlas Project website (https://map.ox.ac.uk /explorer/#). The susceptibility test data is available to download (https://doi.org/10.1101/582510 [8]). Sets of susceptibility test data and predictor variable data in the form used by the statistical modelling analyses are available from GitHub. Numerical data corresponding to the manuscript figures are available to download from Figshare (10.6084/m9.figshare.9912623). R code for implementing the extreme gradient boosting, random forest, and boosted generalized additive models and the R-INLA geostatistical models is available on GitHub at 10.5281/zenodo.3751786 (Hancock, 2020). Funding: This work was funded by Wellcome Trust Grant 108440/Z/15/Z (CLM). The funders had no role in study design, data collection and analysis, decision to publish, or preparation of the manuscript. Competing interests: The authors have declared that no competing interests exist. Abbreviations: BGAM, boosted generalized additive model; CI, credible interval; GMRF, Gaussian Markov random field; IHS, inverse hyperbolic sine; IRS, indoor residual spraying; ITN, insecticide-treated net; LLIN, long-lasting insecticide-treated net; MAE, mean absolute error; PET, potential evapotranspiration; PIT, probability integral transform; RF, random forest model; RMSE, root mean square error; Vgsc, Voltage-gated sodium channel; XGB, extreme gradient boosting model Introduction Insecticide resistance in African malaria vector populations has serious consequences for malaria prevention. Long-lasting insecticide-treated nets (LLINS) have achieved substantial reductions in malaria prevalence thus far in Africa [1], but the number of insecticides currently available for use in LLINS is very limited. Until recently, pyrethroids were the only class approved for use in LLINS, and recently launched new generation nets still use pyrethroids in combination with either an insect growth regulator, a pyrrole, or a synergist that inhibits the primary metabolic mechanism of pyrethroid resistance within mosquitoes [2,3]. A wider range of options is available for indoor residual spraying (IRS), but pyrethroids are less expensive than many alternatives and are still used for IRS in malaria-endemic sub-Saharan African countries [4,5]. Although there is evidence that pyrethroid resistance in African malaria vector populations is increasing [6,7], the wide array of field studies that are available do not provide a spatially comprehensive time series of resistance trends [8]. Quantifying these trends will improve our understanding of the historical spread of resistance and assist in designing insecticide resistance management strategies [9]. Comprehensive spatiotemporal analyses of resistance are also necessary to facilitate its inclusion in epidemiological 9/1/2020, 12:48 PM
Microtubule motors in mitosis David J. Sharp, Gregory C. Rogers & Jonathan M. Scholey Section of Molecular and Cellular Biology, University of California-Davis, I Shields Ave, Davis, California 95616, USA The mitotic spindle uses microtubule-based motor proteins to assemble itself and to segregate sister chromatids. It is becoming clear that motors invoke several distinct mechanisms to generate the forces that drive mitosis. Moreover, in carrying out its function, the spindle appears to pass through a series of transient steady-state structures, each established by a delicate balance of forces generated by multiple complementary and antagonistic motors. Transitions from one steady state to the next can occur when a change in the activity of a subset of mitotic motors tips the balance. T he mitotic spindle is a bipolar, self-organizing microtubule (MT)-based machine that uses energy liberated from nucleotide hydrolysis to segregate sister chromatids accurately into the daughter cells during cell division¹². In this review we focus on two inter-related aspects of spindle action: (1) spindle morphogenesis, characterized by the separation of the spindle poles that occurs during spindle assembly, maintenance and elongation, and (2) chromosome movements that occur during the capture of chromosomes, their alignment on the spindle equator and the segregation of sister chromatids (Fig. 1). Mitotic-spindle function and design The mechanism of spindle action has long fascinated biologists and during the past 50 years, two critical advances were made. First, the observation that the spindle is a very dynamic structure, superficially resembling a liquid crystal', led to the discovery that spindle MTs dis- play dynamic instability, undergoing transitions between episodes of polymerization and depolymerization that are coupled to GTP hydrolysis'. The dynamic properties of MTs are obviously important for spindle morphogenesis as well as force generation within the spindle. Second, the proposal that mitotic movements could be explained by a 'sliding filament' mechanism' led to the elucidation of the structural organization of spindle MTs (Fig. 2) and a search for mitotic motors that could use ATP hydrolysis to drive the sliding of MTs relative to adjacent MTs or to other spindle structures. These motors form the focus of this review. MT-based motor proteins form two families of ATP-dependent force-generating enzymes, the kinesins and the dyneins". Following the seminal discovery of two kinesin motors with mitotic func- tions it has become established that a strikingly large number of MT-based motors participate in spindle action (Table 1). During the past decade, great strides have been made in our understanding of the basic motor mechanisms of mitosis. Surprisingly, it appears that mitotic motors use at least three distinct mechanisms: (1) cross- bridging and sliding MTs relative to adjacent MTs or other structures; (2) transporting specific mitotic cargoes along the surface lattice of spindle MTs; and (3) regulating MT assembly dynamics and cou- pling movement to MT growth and shrinkage (see Box 1). In addi- tion, analyses of the functional inter-relationships between multiple mitotic motors have revealed that specific mitotic movements are not driven by individual motors but, instead, result from shifts in a dynamic balance of complementary and antagonistic forces generat- ed by multiple motors functioning co-operatively. Mitotic motor mechanisms MT sliding. It has long been proposed that force is generated in the spindle by motor-driven MT sliding". For example, motors could crosslink and slide overlapping antiparallel MTs within interpolar MT bundles to position spindle poles in relation to one another. A growing body of evidence indicates that motors actually use two dis- NATURE VOL 407|7 SEPTEMBER 2000 | www.nature.com KEY Microtubule Chromosome arm Centriole Kinetochore Figure 1 Spindle pole and chromosome movements during mitosis. The activities of MTs and their associated motors are responsible for the carefully orchestrated sequence of movements that underlie mitosis. Early in mitosis (prophase and prometaphase) the bipolar spindle assembles (a) and condensed chromosomes are captured by spindle MTs and congress at the spindle equator (d). By metaphase, the spindle consists of two partially interdigitating arrays of MTs emanating from the duplicated centrosomes that form the two spindle poles (b). Chromosomes lie on the equator with pairs of identical sister chromatids facing opposite spindle poles (e). Chromatid segregation involves both the movement of sister chromatids to opposite spindle poles (t; anaphase A) and the elongation of the spindle itself (c; anaphase B). Mechanistically it is convenient to think of these events in terms of spindle assembly. maintenance and elongation (left), and chromosome capture, congression and segregation (right). MT dynamics and length adjustments are in refs 2,5. 2000 Macmillan Magazines Ltd 41 Mapping trends in insecticide resistance phenotypes in African malaria v... https://journals.plos.org/plosbiology/article?id=10.1371/journal.pbio.30... 1 of 17 Mapping trends in insecticide resistance phenotypes in African malaria vectors Penelope A. Hancock, Chantal J. M. Hendriks, Julie-Anne Tangena, Harry Gibson, Janet Hemingway, Michael Coleman, Peter W. Gething, Ewan Cameron, Samir Bhatt, Catherine L. Moyes Published: June 25, 2020 • https://doi.org/10.1371/journal.pbio.3000633 Abstract Mitigating the threat of insecticide resistance in African malaria vector populations requires comprehensive information about where resistance occurs, to what degree, and how this has changed over time. Estimating these trends is complicated by the sparse, heterogeneous distribution of observations of resistance phenotypes in field populations. We use 6,423 observations of the prevalence of resistance to the most important vector control insecticides to inform a Bayesian geostatistical ensemble modelling approach, generating fine-scale predictive maps of resistance phenotypes in mosquitoes from the Anopheles gambiae complex across Africa. Our models are informed by a suite of 111 predictor variables describing potential drivers of selection for resistance. Our maps show alarming increases in the prevalence of resistance to pyrethroids and DDT across sub-Saharan Africa from 2005 to 2017, with mean mortality following insecticide exposure declining from almost 100% to less than 30% in some areas, as well as substantial spatial variation in resistance trends. Citation: Hancock PA, Hendriks CJM, Tangena J-A, Gibson H, Hemingway J, Coleman M, et al. (2020) Mapping trends in insecticide resistance phenotypes in African malaria vectors. PLoS Biol 18(6):e3000633. https://doi.org/10.1371 /journal.pbio.3000633 Academic Editor: Andrew Fraser Read, The Pennsylvania State University, UNITED STATES Received: December 16, 2019; Accepted: May 11, 2020; Published: June 25, 2020 Copyright: ©2020 Hancock et al. This is an open access article distributed under the terms of the Creative Commons Attribution License, which permits unrestricted use, distribution, and reproduction in any medium, provided the original author and source are credited. Data Availability: The predictive maps of the mean prevalence of resistance are available to download from Figshare (10.6084/m9.figshare.9912623) and will be available to visualise on the Malaria Atlas Project website (https://map.ox.ac.uk /explorer/#). The susceptibility test data is available to download (https://doi.org/10.1101/582510 [8]). Sets of susceptibility test data and predictor variable data in the form used by the statistical modelling analyses are available from GitHub. Numerical data corresponding to the manuscript figures are available to download from Figshare (10.6084/m9.figshare.9912623). R code for implementing the extreme gradient boosting, random forest, and boosted generalized additive models and the R-INLA geostatistical models is available on GitHub at 10.5281/zenodo.3751786 (Hancock, 2020). Funding: This work was funded by Wellcome Trust Grant 108440/Z/15/Z (CLM). The funders had no role in study design, data collection and analysis, decision to publish, or preparation of the manuscript. Competing interests: The authors have declared that no competing interests exist. Abbreviations: BGAM, boosted generalized additive model; CI, credible interval; GMRF, Gaussian Markov random field; IHS, inverse hyperbolic sine; IRS, indoor residual spraying; ITN, insecticide-treated net; LLIN, long-lasting insecticide-treated net; MAE, mean absolute error; PET, potential evapotranspiration; PIT, probability integral transform; RF, random forest model; RMSE, root mean square error; Vgsc, Voltage-gated sodium channel; XGB, extreme gradient boosting model Introduction Insecticide resistance in African malaria vector populations has serious consequences for malaria prevention. Long-lasting insecticide-treated nets (LLINS) have achieved substantial reductions in malaria prevalence thus far in Africa [1], but the number of insecticides currently available for use in LLINS is very limited. Until recently, pyrethroids were the only class approved for use in LLINS, and recently launched new generation nets still use pyrethroids in combination with either an insect growth regulator, a pyrrole, or a synergist that inhibits the primary metabolic mechanism of pyrethroid resistance within mosquitoes [2,3]. A wider range of options is available for indoor residual spraying (IRS), but pyrethroids are less expensive than many alternatives and are still used for IRS in malaria-endemic sub-Saharan African countries [4,5]. Although there is evidence that pyrethroid resistance in African malaria vector populations is increasing [6,7], the wide array of field studies that are available do not provide a spatially comprehensive time series of resistance trends [8]. Quantifying these trends will improve our understanding of the historical spread of resistance and assist in designing insecticide resistance management strategies [9]. Comprehensive spatiotemporal analyses of resistance are also necessary to facilitate its inclusion in epidemiological 9/1/2020, 12:48 PM
Biology: The Dynamic Science (MindTap Course List)
4th Edition
ISBN:9781305389892
Author:Peter J. Russell, Paul E. Hertz, Beverly McMillan
Publisher:Peter J. Russell, Paul E. Hertz, Beverly McMillan
Chapter10: Cell Division And Mitosis
Section: Chapter Questions
Problem 1ITD: Biologists have long been interested in the effects of radiation on cells. In one experiment,...
Related questions
Question
a) What is the title of the original research article?
b) What clues tell you which is a review and which is an original research paper?

Transcribed Image Text:Microtubule motors in mitosis
David J. Sharp, Gregory C. Rogers & Jonathan M. Scholey
Section of Molecular and Cellular Biology, University of California-Davis, I Shields Ave, Davis, California 95616, USA
The mitotic spindle uses microtubule-based motor proteins to assemble itself and to segregate sister chromatids. It is
becoming clear that motors invoke several distinct mechanisms to generate the forces that drive mitosis. Moreover, in
carrying out its function, the spindle appears to pass through a series of transient steady-state structures, each established
by a delicate balance of forces generated by multiple complementary and antagonistic motors. Transitions from one steady
state to the next can occur when a change in the activity of a subset of mitotic motors tips the balance.
T
he mitotic spindle is a bipolar, self-organizing
microtubule (MT)-based machine that uses energy
liberated from nucleotide hydrolysis to segregate sister
chromatids accurately into the daughter cells during cell
division¹². In this review we focus on two inter-related
aspects of spindle action: (1) spindle morphogenesis, characterized
by the separation of the spindle poles that occurs during spindle
assembly, maintenance and elongation, and (2) chromosome
movements that occur during the capture of chromosomes, their
alignment on the spindle equator and the segregation of sister
chromatids (Fig. 1).
Mitotic-spindle function and design
The mechanism of spindle action has long fascinated biologists and
during the past 50 years, two critical advances were made. First, the
observation that the spindle is a very dynamic structure, superficially
resembling a liquid crystal', led to the discovery that spindle MTs dis-
play dynamic instability, undergoing transitions between episodes of
polymerization and depolymerization that are coupled to GTP
hydrolysis'. The dynamic properties of MTs are obviously important
for spindle morphogenesis as well as force generation within the
spindle. Second, the proposal that mitotic movements could be
explained by a 'sliding filament' mechanism' led to the elucidation of
the structural organization of spindle MTs (Fig. 2) and a search for
mitotic motors that could use ATP hydrolysis to drive the sliding of
MTs relative to adjacent MTs or to other spindle structures. These
motors form the focus of this review.
MT-based motor proteins form two families of ATP-dependent
force-generating enzymes, the kinesins and the dyneins". Following
the seminal discovery of two kinesin motors with mitotic func-
tions it has become established that a strikingly large number of
MT-based motors participate in spindle action (Table 1). During the
past decade, great strides have been made in our understanding of the
basic motor mechanisms of mitosis. Surprisingly, it appears that
mitotic motors use at least three distinct mechanisms: (1) cross-
bridging and sliding MTs relative to adjacent MTs or other structures;
(2) transporting specific mitotic cargoes along the surface lattice of
spindle MTs; and (3) regulating MT assembly dynamics and cou-
pling movement to MT growth and shrinkage (see Box 1). In addi-
tion, analyses of the functional inter-relationships between multiple
mitotic motors have revealed that specific mitotic movements are
not driven by individual motors but, instead, result from shifts in a
dynamic balance of complementary and antagonistic forces generat-
ed by multiple motors functioning co-operatively.
Mitotic motor mechanisms
MT sliding. It has long been proposed that force is generated in the
spindle by motor-driven MT sliding". For example, motors could
crosslink and slide overlapping antiparallel MTs within interpolar
MT bundles to position spindle poles in relation to one another. A
growing body of evidence indicates that motors actually use two dis-
NATURE VOL 407|7 SEPTEMBER 2000 | www.nature.com
KEY
Microtubule
Chromosome
arm
Centriole
Kinetochore
Figure 1 Spindle pole and chromosome movements during mitosis. The activities of
MTs and their associated motors are responsible for the carefully orchestrated
sequence of movements that underlie mitosis. Early in mitosis (prophase and
prometaphase) the bipolar spindle assembles (a) and condensed chromosomes are
captured by spindle MTs and congress at the spindle equator (d). By metaphase, the
spindle consists of two partially interdigitating arrays of MTs emanating from the
duplicated centrosomes that form the two spindle poles (b). Chromosomes lie on the
equator with pairs of identical sister chromatids facing opposite spindle poles (e).
Chromatid segregation involves both the movement of sister chromatids to opposite
spindle poles (t; anaphase A) and the elongation of the spindle itself (c; anaphase B).
Mechanistically it is convenient to think of these events in terms of spindle assembly.
maintenance and elongation (left), and chromosome capture, congression and
segregation (right). MT dynamics and length adjustments are in refs 2,5.
2000 Macmillan Magazines Ltd
41
![Mapping trends in insecticide resistance phenotypes in African malaria v... https://journals.plos.org/plosbiology/article?id=10.1371/journal.pbio.30...
1 of 17
Mapping trends in insecticide resistance phenotypes in
African malaria vectors
Penelope A. Hancock, Chantal J. M. Hendriks, Julie-Anne Tangena, Harry Gibson, Janet Hemingway, Michael Coleman,
Peter W. Gething, Ewan Cameron, Samir Bhatt, Catherine L. Moyes
Published: June 25, 2020 • https://doi.org/10.1371/journal.pbio.3000633
Abstract
Mitigating the threat of insecticide resistance in African malaria vector populations requires comprehensive information about where
resistance occurs, to what degree, and how this has changed over time. Estimating these trends is complicated by the sparse,
heterogeneous distribution of observations of resistance phenotypes in field populations. We use 6,423 observations of the
prevalence of resistance to the most important vector control insecticides to inform a Bayesian geostatistical ensemble modelling
approach, generating fine-scale predictive maps of resistance phenotypes in mosquitoes from the Anopheles gambiae complex
across Africa. Our models are informed by a suite of 111 predictor variables describing potential drivers of selection for resistance.
Our maps show alarming increases in the prevalence of resistance to pyrethroids and DDT across sub-Saharan Africa from 2005 to
2017, with mean mortality following insecticide exposure declining from almost 100% to less than 30% in some areas, as well as
substantial spatial variation in resistance trends.
Citation: Hancock PA, Hendriks CJM, Tangena J-A, Gibson H, Hemingway J, Coleman M, et al. (2020) Mapping trends in
insecticide resistance phenotypes in African malaria vectors. PLoS Biol 18(6):e3000633. https://doi.org/10.1371
/journal.pbio.3000633
Academic Editor: Andrew Fraser Read, The Pennsylvania State University, UNITED STATES
Received: December 16, 2019; Accepted: May 11, 2020; Published: June 25, 2020
Copyright: ©2020 Hancock et al. This is an open access article distributed under the terms of the Creative Commons
Attribution License, which permits unrestricted use, distribution, and reproduction in any medium, provided the original author
and source are credited.
Data Availability: The predictive maps of the mean prevalence of resistance are available to download from Figshare
(10.6084/m9.figshare.9912623) and will be available to visualise on the Malaria Atlas Project website (https://map.ox.ac.uk
/explorer/#). The susceptibility test data is available to download (https://doi.org/10.1101/582510 [8]). Sets of susceptibility
test data and predictor variable data in the form used by the statistical modelling analyses are available from GitHub.
Numerical data corresponding to the manuscript figures are available to download from Figshare
(10.6084/m9.figshare.9912623). R code for implementing the extreme gradient boosting, random forest, and boosted
generalized additive models and the R-INLA geostatistical models is available on GitHub at 10.5281/zenodo.3751786
(Hancock, 2020).
Funding: This work was funded by Wellcome Trust Grant 108440/Z/15/Z (CLM). The funders had no role in study design,
data collection and analysis, decision to publish, or preparation of the manuscript.
Competing interests: The authors have declared that no competing interests exist.
Abbreviations: BGAM, boosted generalized additive model; CI, credible interval; GMRF, Gaussian Markov random field;
IHS, inverse hyperbolic sine; IRS, indoor residual spraying; ITN, insecticide-treated net; LLIN, long-lasting insecticide-treated
net; MAE, mean absolute error; PET, potential evapotranspiration; PIT, probability integral transform; RF, random forest
model; RMSE, root mean square error; Vgsc, Voltage-gated sodium channel; XGB, extreme gradient boosting model
Introduction
Insecticide resistance in African malaria vector populations has serious consequences for malaria prevention. Long-lasting
insecticide-treated nets (LLINS) have achieved substantial reductions in malaria prevalence thus far in Africa [1], but the number of
insecticides currently available for use in LLINS is very limited. Until recently, pyrethroids were the only class approved for use in
LLINS, and recently launched new generation nets still use pyrethroids in combination with either an insect growth regulator, a
pyrrole, or a synergist that inhibits the primary metabolic mechanism of pyrethroid resistance within mosquitoes [2,3]. A wider range
of options is available for indoor residual spraying (IRS), but pyrethroids are less expensive than many alternatives and are still
used for IRS in malaria-endemic sub-Saharan African countries [4,5].
Although there is evidence that pyrethroid resistance in African malaria vector populations is increasing [6,7], the wide array of field
studies that are available do not provide a spatially comprehensive time series of resistance trends [8]. Quantifying these trends will
improve our understanding of the historical spread of resistance and assist in designing insecticide resistance management
strategies [9]. Comprehensive spatiotemporal analyses of resistance are also necessary to facilitate its inclusion in epidemiological
9/1/2020, 12:48 PM](/v2/_next/image?url=https%3A%2F%2Fcontent.bartleby.com%2Fqna-images%2Fquestion%2F3cff6d5e-6d7b-4ef6-9838-a544f8e2903b%2F36fa434f-1486-4193-becd-e9e16cd6815a%2F3j0qlwf_processed.png&w=3840&q=75)
Transcribed Image Text:Mapping trends in insecticide resistance phenotypes in African malaria v... https://journals.plos.org/plosbiology/article?id=10.1371/journal.pbio.30...
1 of 17
Mapping trends in insecticide resistance phenotypes in
African malaria vectors
Penelope A. Hancock, Chantal J. M. Hendriks, Julie-Anne Tangena, Harry Gibson, Janet Hemingway, Michael Coleman,
Peter W. Gething, Ewan Cameron, Samir Bhatt, Catherine L. Moyes
Published: June 25, 2020 • https://doi.org/10.1371/journal.pbio.3000633
Abstract
Mitigating the threat of insecticide resistance in African malaria vector populations requires comprehensive information about where
resistance occurs, to what degree, and how this has changed over time. Estimating these trends is complicated by the sparse,
heterogeneous distribution of observations of resistance phenotypes in field populations. We use 6,423 observations of the
prevalence of resistance to the most important vector control insecticides to inform a Bayesian geostatistical ensemble modelling
approach, generating fine-scale predictive maps of resistance phenotypes in mosquitoes from the Anopheles gambiae complex
across Africa. Our models are informed by a suite of 111 predictor variables describing potential drivers of selection for resistance.
Our maps show alarming increases in the prevalence of resistance to pyrethroids and DDT across sub-Saharan Africa from 2005 to
2017, with mean mortality following insecticide exposure declining from almost 100% to less than 30% in some areas, as well as
substantial spatial variation in resistance trends.
Citation: Hancock PA, Hendriks CJM, Tangena J-A, Gibson H, Hemingway J, Coleman M, et al. (2020) Mapping trends in
insecticide resistance phenotypes in African malaria vectors. PLoS Biol 18(6):e3000633. https://doi.org/10.1371
/journal.pbio.3000633
Academic Editor: Andrew Fraser Read, The Pennsylvania State University, UNITED STATES
Received: December 16, 2019; Accepted: May 11, 2020; Published: June 25, 2020
Copyright: ©2020 Hancock et al. This is an open access article distributed under the terms of the Creative Commons
Attribution License, which permits unrestricted use, distribution, and reproduction in any medium, provided the original author
and source are credited.
Data Availability: The predictive maps of the mean prevalence of resistance are available to download from Figshare
(10.6084/m9.figshare.9912623) and will be available to visualise on the Malaria Atlas Project website (https://map.ox.ac.uk
/explorer/#). The susceptibility test data is available to download (https://doi.org/10.1101/582510 [8]). Sets of susceptibility
test data and predictor variable data in the form used by the statistical modelling analyses are available from GitHub.
Numerical data corresponding to the manuscript figures are available to download from Figshare
(10.6084/m9.figshare.9912623). R code for implementing the extreme gradient boosting, random forest, and boosted
generalized additive models and the R-INLA geostatistical models is available on GitHub at 10.5281/zenodo.3751786
(Hancock, 2020).
Funding: This work was funded by Wellcome Trust Grant 108440/Z/15/Z (CLM). The funders had no role in study design,
data collection and analysis, decision to publish, or preparation of the manuscript.
Competing interests: The authors have declared that no competing interests exist.
Abbreviations: BGAM, boosted generalized additive model; CI, credible interval; GMRF, Gaussian Markov random field;
IHS, inverse hyperbolic sine; IRS, indoor residual spraying; ITN, insecticide-treated net; LLIN, long-lasting insecticide-treated
net; MAE, mean absolute error; PET, potential evapotranspiration; PIT, probability integral transform; RF, random forest
model; RMSE, root mean square error; Vgsc, Voltage-gated sodium channel; XGB, extreme gradient boosting model
Introduction
Insecticide resistance in African malaria vector populations has serious consequences for malaria prevention. Long-lasting
insecticide-treated nets (LLINS) have achieved substantial reductions in malaria prevalence thus far in Africa [1], but the number of
insecticides currently available for use in LLINS is very limited. Until recently, pyrethroids were the only class approved for use in
LLINS, and recently launched new generation nets still use pyrethroids in combination with either an insect growth regulator, a
pyrrole, or a synergist that inhibits the primary metabolic mechanism of pyrethroid resistance within mosquitoes [2,3]. A wider range
of options is available for indoor residual spraying (IRS), but pyrethroids are less expensive than many alternatives and are still
used for IRS in malaria-endemic sub-Saharan African countries [4,5].
Although there is evidence that pyrethroid resistance in African malaria vector populations is increasing [6,7], the wide array of field
studies that are available do not provide a spatially comprehensive time series of resistance trends [8]. Quantifying these trends will
improve our understanding of the historical spread of resistance and assist in designing insecticide resistance management
strategies [9]. Comprehensive spatiotemporal analyses of resistance are also necessary to facilitate its inclusion in epidemiological
9/1/2020, 12:48 PM
Expert Solution

This question has been solved!
Explore an expertly crafted, step-by-step solution for a thorough understanding of key concepts.
Step by step
Solved in 2 steps

Recommended textbooks for you
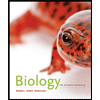
Biology: The Dynamic Science (MindTap Course List)
Biology
ISBN:
9781305389892
Author:
Peter J. Russell, Paul E. Hertz, Beverly McMillan
Publisher:
Cengage Learning
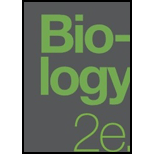
Biology 2e
Biology
ISBN:
9781947172517
Author:
Matthew Douglas, Jung Choi, Mary Ann Clark
Publisher:
OpenStax
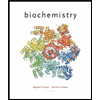
Biochemistry
Biochemistry
ISBN:
9781305577206
Author:
Reginald H. Garrett, Charles M. Grisham
Publisher:
Cengage Learning
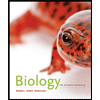
Biology: The Dynamic Science (MindTap Course List)
Biology
ISBN:
9781305389892
Author:
Peter J. Russell, Paul E. Hertz, Beverly McMillan
Publisher:
Cengage Learning
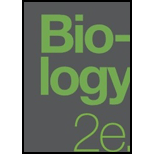
Biology 2e
Biology
ISBN:
9781947172517
Author:
Matthew Douglas, Jung Choi, Mary Ann Clark
Publisher:
OpenStax
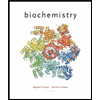
Biochemistry
Biochemistry
ISBN:
9781305577206
Author:
Reginald H. Garrett, Charles M. Grisham
Publisher:
Cengage Learning
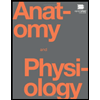
Anatomy & Physiology
Biology
ISBN:
9781938168130
Author:
Kelly A. Young, James A. Wise, Peter DeSaix, Dean H. Kruse, Brandon Poe, Eddie Johnson, Jody E. Johnson, Oksana Korol, J. Gordon Betts, Mark Womble
Publisher:
OpenStax College
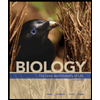
Biology: The Unity and Diversity of Life (MindTap…
Biology
ISBN:
9781337408332
Author:
Cecie Starr, Ralph Taggart, Christine Evers, Lisa Starr
Publisher:
Cengage Learning
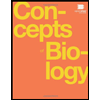
Concepts of Biology
Biology
ISBN:
9781938168116
Author:
Samantha Fowler, Rebecca Roush, James Wise
Publisher:
OpenStax College