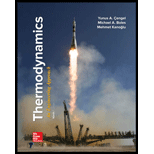
A refrigerator operating on the vapor-compression refrigeration cycle using refrigerant-134a as the refrigerant is considered. The temperatures of the cooled space and the ambient air are at 10°F and 80°F, respectively. R-134a enters the compressor at 20 psia as a saturated vapor and leaves at 140 psia and 160°F. The refrigerant leaves the condenser as a saturated liquid. The rate of cooling provided by the system is 45,000 Btu/h. Determine (a) the mass flow rate of R-134a and the COP, (b) the exergy destruction in each component of the cycle and the second-law efficiency of the compressor, and (c) the second-law efficiency of the cycle and the total exergy destruction in the cycle.
(a)

The mass flow rate of R-134a and the COP.
Answer to Problem 33P
The mass flow rate of R-134a and the COP is
Explanation of Solution
Show the T-s diagram for vapor-compression refrigeration cycle as in Figure (1).
From Figure (1), write the specific enthalpy at state 3 is equal to state 4 due to throttling process.
Here, specific enthalpy at state 3 and 4 is
Express the work input.
Here, specific enthalpy at state 2 and 1 is
Express heat supplied to the cooled space.
Express the heat removed from the cooled space.
Express quality at state 4.
Here, specific enthalpy at saturated liquid and evaporation and
Express specific entropy at state 4.
Here, specific entropy at saturated liquid and evaporation and
Express mass flow rate of R-134a.
Here, rate of heat lost is
Express the COP of the cycle.
Conclusion:
Refer Table A-12E, “saturated refrigerant-134a-pressure table”, and write the properties corresponding to initial pressure
Here, specific entropy at state 1 is
Refer Table A-13E, “superheated refrigerant-134a”, and write the properties corresponding to pressure at state 2
Here, specific entropy at state 2 is
Refer Table A-12E, “saturated refrigerant-134a-pressure table”, and write the properties corresponding to pressure at state 3
Here, specific entropy at state 3 is
As specific enthalpy at state 3 is equal to specific enthalpy at state 4,
Refer Table A-12E, “saturated refrigerant-134a-pressure table”, and write the properties corresponding to pressure at state 4
Substitute
Substitute
Substitute
Substitute
Substitute
Substitute
Substitute
Hence, the mass flow rate of R-134a and the COP is
(b)

The exergy destruction in each component of the cycle and the second-law efficiency of the compressor.
Answer to Problem 33P
The exergy destruction in compressor is
Explanation of Solution
For compressor:
Express the exergy destruction in compressor.
Here, surrounding temperature is
For condenser:
Express the exergy destruction in condenser.
Here, entropy generation during process 2-3 is
For expansion valve:
For evaporator:
Express the exergy destruction in evaporator.
Here, entropy generation during process 4-1 is
Express the power input of the compressor.
Express second law efficiency of the compressor.
Conclusion:
Perform unit conversion of surrounding temperature from
Perform unit conversion of high temperature medium from
Perform unit conversion of low temperature medium from
Substitute
Hence, the exergy destruction in compressor is
Substitute
Hence, the exergy destruction in condenser is
Substitute
Hence, the exergy destruction in expansion valve is
Substitute
Hence, the exergy destruction in evaporator is
Substitute
Substitute
Hence, the second-law efficiency of the compressor is
(c)

The second-law efficiency of the cycle and the total exergy destruction in the cycle.
Answer to Problem 33P
The second-law efficiency of the cycle is
Explanation of Solution
Express the exergy of the heat transferred from the low temperature medium.
Determine the second law efficiency of the cycle.
Express the total exergy destruction in the cycle.
Conclusion:
Substitute
Substitute
Hence, the second-law efficiency of the cycle is
Substitute
Hence, the total exergy destruction in the cycle is
Want to see more full solutions like this?
Chapter 11 Solutions
Thermodynamics: An Engineering Approach
- الثانية Babakt Momentum equation for Boundary Layer S SS -Txfriction dray Momentum equation for Boundary Layer What laws are important for resolving issues 2 How to draw. 3 What's Point about this.arrow_forwardR αι g The system given on the left, consists of three pulleys and the depicted vertical ropes. Given: ri J₁, m1 R = 2r; απ r2, J2, m₂ m1; m2; M3 J1 J2 J3 J3, m3 a) Determine the radii 2 and 3.arrow_forwardB: Solid rotating shaft used in the boat with high speed shown in Figure. The amount of power transmitted at the greatest torque is 224 kW with 130 r.p.m. Used DE-Goodman theory to determine the shaft diameter. Take the shaft material is annealed AISI 1030, the endurance limit of 18.86 kpsi and a factor of safety 1. Which criterion is more conservative? Note: all dimensions in mm. 1 AA Motor 300 Thrust Bearing Sprocket 100 9750 เอarrow_forward
- Q2: The plate material of a pressure vessel is AISI 1050 QT 205 °C. The plate is rolled to a diameter of 1.2 m. The two sides of the plate are connected via a riveted joint as shown below. If the rivet material is G10500 with HB=197 and all rivet sizes M31. Find the required rivet size when the pressure vessel is subjected to an internal pressure of 500 MPa. Take safety factor = 2. 1.2m A B' A Chope olm 10.5 0.23 hopearrow_forwardContinuity equation A y x dx D T معادلة الاستمرارية Ly X Q/Prove that ди хе + ♥+ ㅇ? he me ze ོ༞“༠ ?arrow_forwardQ Derive (continuity equation)? I want to derive clear mathematics.arrow_forward
- motor supplies 200 kW at 6 Hz to flange A of the shaft shown in Figure. Gear B transfers 125 W of power to operating machinery in the factory, and the remaining power in the shaft is mansferred by gear D. Shafts (1) and (2) are solid aluminum (G = 28 GPa) shafts that have the same diameter and an allowable shear stress of t= 40 MPa. Shaft (3) is a solid steel (G = 80 GPa) shaft with an allowable shear stress of t = 55 MPa. Determine: a) the minimum permissible diameter for aluminum shafts (1) and (2) b) the minimum permissible diameter for steel shaft (3). c) the rotation angle of gear D with respect to flange A if the shafts have the minimum permissible diameters as determined in (a) and (b).arrow_forwardFirst monthly exam Gas dynamics Third stage Q1/Water at 15° C flow through a 300 mm diameter riveted steel pipe, E-3 mm with a head loss of 6 m in 300 m length. Determine the flow rate in pipe. Use moody chart. Q2/ Assume a car's exhaust system can be approximated as 14 ft long and 0.125 ft-diameter cast-iron pipe ( = 0.00085 ft) with the equivalent of (6) regular 90° flanged elbows (KL = 0.3) and a muffler. The muffler acts as a resistor with a loss coefficient of KL= 8.5. Determine the pressure at the beginning of the exhaust system (pl) if the flowrate is 0.10 cfs, and the exhaust has the same properties as air.(p = 1.74 × 10-3 slug/ft³, u= 4.7 x 10-7 lb.s/ft²) Use moody chart (1) MIDAS Kel=0.3 Q3/Liquid ammonia at -20°C is flowing through a 30 m long section of a 5 mm diameter copper tube(e = 1.5 × 10-6 m) at a rate of 0.15 kg/s. Determine the pressure drop and the head losses. .μ= 2.36 × 10-4 kg/m.s)p = 665.1 kg/m³arrow_forward2/Y Y+1 2Cp Q1/ Show that Cda Az x P1 mactual Cdf Af R/T₁ 2pf(P1-P2-zxgxpf) Q2/ A simple jet carburetor has to supply 5 Kg of air per minute. The air is at a pressure of 1.013 bar and a temperature of 27 °C. Calculate the throat diameter of the choke for air flow velocity of 90 m/sec. Take velocity coefficient to be 0.8. Assume isentropic flow and the flow to be compressible. Quiz/ Determine the air-fuel ratio supplied at 5000 m altitude by a carburetor which is adjusted to give an air-fuel ratio of 14:1 at sea level where air temperature is 27 °C and pressure is 1.013 bar. The temperature of air decreases with altitude as given by the expression The air pressure decreases with altitude as per relation h = 19200 log10 (1.013), where P is in bar. State any assumptions made. t = ts P 0.0065harrow_forward
- 36 2) Use the method of MEMBERS to determine the true magnitude and direction of the forces in members1 and 2 of the frame shown below in Fig 3.2. 300lbs/ft member-1 member-2 30° Fig 3.2. https://brightspace.cuny.edu/d21/le/content/433117/viewContent/29873977/Viewarrow_forwardCan you solve this for me?arrow_forward5670 mm The apartment in the ground floor of three floors building in Fig. in Baghdad city. The details of walls, roof, windows and door are shown. The window is a double glazing and air space thickness is 1.3cm Poorly Fitted-with Storm Sash with wood strip and storm window of 0.6 cm glass thickness. The thickness of door is 2.5 cm. The door is Poor Installation. There are two peoples in each room. The height of room is 280 cm. assume the indoor design conditions are 25°C DBT and 50 RH, and moisture content of 8 gw/kga. The moisture content of outdoor is 10.5 gw/kga. Calculate heat gain for living room : الشقة في الطابق الأرضي من مبنى ثلاثة طوابق في مدينة بغداد يظهر في مخطط الشقة تفاصيل الجدران والسقف والنوافذ والباب. النافذة عبارة عن زجاج مزدوج وسمك الفراغ الهوائي 1.3 سم ضعيف الاحكام مع ساتر حماية مع إطار خشبي والنافذة بسماكة زجاج 0.6 سم سماكة الباب 2.5 سم. الباب هو تركيب ضعيف هناك شخصان في كل غرفة. ارتفاع الغرفة 280 سم. افترض أن ظروف التصميم الداخلي هي DBT25 و R50 ، ومحتوى الرطوبة 8…arrow_forward
- Refrigeration and Air Conditioning Technology (Mi...Mechanical EngineeringISBN:9781305578296Author:John Tomczyk, Eugene Silberstein, Bill Whitman, Bill JohnsonPublisher:Cengage Learning
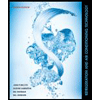