
Reconsider Prob. 10–83. Determine which components of the combined cycle are the most wasteful of work potential.
10–83 A combined gas–steam power cycle uses a simple gas turbine for the topping cycle and simple Rankine cycle for the bottoming cycle. Atmospheric air enters the gas turbine at 101 kPa and 20°C, and the maximum gas cycle temperature is 1100°C. The compressor pressure ratio is 8; the compressor isentropic efficiency is 85 percent; and the gas turbine isentropic efficiency is 90 percent. The gas stream leaves the heat exchanger at the saturation temperature of the steam flowing through the heat exchanger. Steam flows through the heat exchanger with a pressure of 6000 kPa and leaves at 320°C. The steam-cycle condenser operates at 20 kPa, and the isentropic efficiency of the steam turbine is 90 percent. Determine the mass flow rate of air through the air compressor required for this system to produce 100 MW of power. Use constant specific heats for air at room temperature.

Which component of the combined cycle is the most wasteful of work potential.
Answer to Problem 85P
The combustor of the gas-steam cycle has largest exergy destruction
Explanation of Solution
Show the
Refer Figure 1.
Consider the gas cycle (topping cycle) and their respective process states such as 5, 6,
Write the temperature and pressure relation at isentropic state and for the process 5-6-
Here, the temperature is
Write the formula for isentropic efficiency of compressor for the process 5-6-
Here, the enthalpy is
Rearrange and rewrite the equation (II) to obtain
Write the temperature and pressure relation at isentropic state and for the process 7-8-
Write the formula for isentropic efficiency of gas turbine
Rearrange and rewrite the equation (V) to obtain
At state 9: (heat exchanger)
The temperature
Refer Table A-5, “Saturated water-Pressure table”.
The saturation temperature corresponding to the pressure of
Refer Figure 1.
Consider the steam cycle (bottoming cycle) and their respective process states such as 1, 2, 3, 4,
At state 1:
The water exits the condenser as a saturated liquid at the pressure of
Refer Table A-5, “Saturated water-Pressure table”.
The enthalpy
At state 2:
Write the formula for work done by the pump during process 1-2.
Here, the specific volume is
Write the formula for enthalpy
At state 3: (Turbine inlet)
The steam enters the turbine as superheated vapour.
Refer Table A-6, “Superheated water”.
The enthalpy
From Figure 1,
At state 4: (Turbine exit or condenser inlet)
The steam exits the condenser as a saturated liquid at the pressure of
The quality of water at the exit of the turbine is expressed as follows.
The enthalpy at state
Here, the enthalpy is
Refer Table A-5, “Saturated water-Pressure table”.
Obtain the following properties corresponding to the pressure of
Write the formula for isentropic efficiency of the steam turbine
Rearrange the Equation (XI) to obtain the enthalpy
Write the formula for net work output of the gas cycle.
Here, the specific heat of air at constant pressure is
Write the formula for net work output of the steam cycle.
Write the general energy rate balance equation.
Here, the rate of energy in is
Consider the heat exchanger operates on steady state. Hence, the rate of change in net energy of the system is zero.
The Equation (XV) is reduced as follows for the heat exchanger.
Here, the mass flow rate of air is
Write the formula for mass flow rate of air through the compressor.
Write the formula for the exergy destruction for the process 3-4 (turbine).
Write the formula for the exergy destruction for the process 4-1 (condenser).
Write the formula for the exergy destruction for heat exchanger.
Write the formula for the exergy destruction for the process 5-6 (compressor).
Write the formula for the exergy destruction for the process 6-7 (combustion chamber).
Write the formula for the exergy destruction for the process 7-8 (gas turbine).
Here, the specific heat at constant pressure of air is
Refer Table A-2, “Ideal-gas specific heats of various common gases”.
The specific heat at constant pressure
Conclusion:
Substitute
Substitute
Substitute
Substitute
Substitute
Substitute
Here,
Substitute
Substitute
Equation (X).
Substitute
Substitute
Substitute
Equation (XIV).
Substitute
When, the mass flow rate of air is
Substitute
Thus, the Equation (XXIV) describes that
Substitute
Thus, the mass flow rate of the air through the air compressor required for this system to produce
Substitute
Consider the process 1 to 2 (Pump).
Here, the pump is isentropic. Hence the exergy destruction during the process 1-2 is zero.
Consider the process 3 to 4 (steam turbine).
Here,
Substitute
Thus, the exergy destruction during process 3-4 is
Substitute
Substitute
Substitute
Substitute
Substitute
The calculated exergy destruction value is greater for component combustor that is
Hence, the combustor of the gas-steam cycle has largest exergy destruction of all other components and that is the most wasteful of work potential.
Want to see more full solutions like this?
Chapter 10 Solutions
Thermodynamics: An Engineering Approach ( 9th International Edition ) ISBN:9781260092684
Additional Engineering Textbook Solutions
Heating Ventilating and Air Conditioning: Analysis and Design
Fluid Mechanics: Fundamentals and Applications
Vector Mechanics for Engineers: Statics and Dynamics
Manufacturing Engineering & Technology
Engineering Mechanics: Statics
Statics and Mechanics of Materials
- 12 Th 04 A- A steam power plant operates on a simple ideal Rankine cycle The steam enters the turbine at pressure of 5 MPa and leaves at 10 kPa and 90% quality. The mass flow rate of steam through the cycle is 35 kg/s. Show the cycle on a T-s diagram and determine (a) the thermal efficiency of the cycle and (b) the net power output of the power plant.arrow_forwardA steam power plant operates on the ideal reheat Rankine cycle. Steam enters the high-pressure turbine at 7 MPa and 450°C and leaves at 2 MPa. Steam is then reheated at constant pressure to 450°C before it expands to 20 kPa in the low-pressure turbine. Determine the turbine work output, in kJ/kg, and the thermal efficiency of the cycle. Also, show the cycle on T-S diagram with respect to saturation lines.arrow_forwardRequired information. The net work output and the thermal efficiency for the Carnot and the simple ideal Rankine cycles with steam as the working fluid are to be calculated and compared. Steam enters the turbine in both cases at 10 MPa as a saturated vapor, and the condenser pressure is 50 kPa. In the Rankine cycle, the condenser exit state is saturated liquid and, in the Carnot cycle, the boiler inlet state is saturated liquid. Draw the T-s diagrams for both cycles. (Please upload your response/solution using the controls below.) upload a response file (15MB max) Choose File no file selected savearrow_forward
- A combined gas-steam power cycle uses a simple gas turbine for the topping cycle and simple Rankine cycle for the bottoming cycle, Atmospheric air enters the gas turbine at 101 kPa and 20°C, and the maximum gas cycle temperature is 1100°C. The compressor pressure ratio is 8, the compressor isentropic efficiency is 85 percent, and the gas turbine isentropic efficiency is 90 percent. The gas stream leaves the heat exchanger at the saturation temperature of the steam flowing through the heat exchanger. Steam flows through the heat exchanger with a pressure of 6000 kPa and leaves at 320°C. The steam-cycle condenser operates at 20 kPa, and the isentropic efficiency of the steam turbine is 90 percent. Determine the mass flow rate of air through the air compressor required for this system to produce 110 MW of power. Use constant specific heats for air at room temperature. Use steam tables and the table containing the ideal-gas specific heats of various common gases. The required mass flow…arrow_forwardI need the answer as soon as possiblearrow_forwardT2arrow_forward
- * A 200 MW Steam Power Plant operates on a simple Rankine Cycle assuming non-ideal isentific efficiencies for the water pump and the steam turbine. The Plant consists of a water pump with isentrafic efficiency 0.9, a baler, a a baler, a turbine with isentrafic efficiency 0.83 and a condenser. The steam exits the boiler at Pressure P₂ = 10MPa and temperature T3 = 500°C. The exit Pressure at the Condenser is 50kPa. i) Sketch the cycle on a T-s diagram Calculate the quality of the stream at the turbine exit lii.) Find the thermal efficiency of the plant iv.) Find the steam mass flow rate. larrow_forward4. Consider a simple ideal Rankine cycle. The pressure of the boiler and the condenser are 3 Mpa and 10 kPa respectively. The temperature of the steam entering the turbine is 350°C, and the quality of mixture leaving the turbine is 0.8. Calculate the back work ratio, in %. 0.4% - 0.5% · 0.3 % - 0.6%arrow_forward2. Consider an ideal gas-turbine cycle with two stages of compression with intercooling. The overall pressure ratio is 9. The air enters each stage of the compressor at 300 K and of the turbine at 1300 K. Utilizing air-standard assumptions, determine the back-work ratio and the thermal efficiency of the cycle. 3. Consider an ideal gas-turbine cycle with two stages of expansion with reheating. The overall pressure ratio is 9. The air the compressor at 300 K and the air enter each stage of the turbine at 1300 K. Utilizing air-standard assumptions, determine the back-work ratio and the thermal efficiency of the cycle.arrow_forward
- Consider a simple Rankine cycle and an ideal Rankine cycle with three reheat stages.Both cycles operate between the same pressure limits. The maximum temperature is700°C in the simple cycle and 450°C in the reheat cycle. Which cycle do you think willhave a higher thermal efficiency?arrow_forwardAnalyze an ideal Otto cycle to obtain it heat rejection, the net work production and the mean effective pressure for each repetition of the cycle. The compression ratio of 7 and at the beginning of the compression process, P1 = 90 kPa, T1 = 27°C, and Vi = 0.004 m³. The maximum cycle temperature is 1127°C. Assume constant specific heats at room temperature.arrow_forwardConsider a steam power plant that operates on a simple ideal Rankine cycle and has a net power output of 120 MW. Steam enters the turbine at 10 MPa and 550°C and is cooled in the condenser at a pressure of 15 kPa by running cooling water from a nearby source the condenser at a rate of 3000 kg/s. The isentropic efficiency of the pump is 95% and hy =226, hy 240 kkg, hg 3520 g, and ha- 2210 kg. Determine the Actual work input to the Compressor 13.6 kj/kg e 14.7 kkg 14.1 kkg 15.2 kkgarrow_forward
- Elements Of ElectromagneticsMechanical EngineeringISBN:9780190698614Author:Sadiku, Matthew N. O.Publisher:Oxford University PressMechanics of Materials (10th Edition)Mechanical EngineeringISBN:9780134319650Author:Russell C. HibbelerPublisher:PEARSONThermodynamics: An Engineering ApproachMechanical EngineeringISBN:9781259822674Author:Yunus A. Cengel Dr., Michael A. BolesPublisher:McGraw-Hill Education
- Control Systems EngineeringMechanical EngineeringISBN:9781118170519Author:Norman S. NisePublisher:WILEYMechanics of Materials (MindTap Course List)Mechanical EngineeringISBN:9781337093347Author:Barry J. Goodno, James M. GerePublisher:Cengage LearningEngineering Mechanics: StaticsMechanical EngineeringISBN:9781118807330Author:James L. Meriam, L. G. Kraige, J. N. BoltonPublisher:WILEY
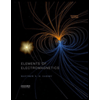
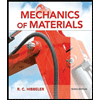
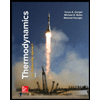
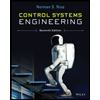
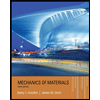
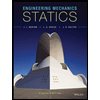