Hoppe_M7_LabReport
docx
keyboard_arrow_up
School
Excelsior University *
*We aren’t endorsed by this school
Course
NUC 323
Subject
Mechanical Engineering
Date
Feb 20, 2024
Type
docx
Pages
17
Uploaded by ProfMusic11383
Course: NUC350 Section: Module 7
Instructor Name: Professor Mathus
Name(s): Zachary Hoppe
__________________________________________________________________________
Title
: Turbine Load Reduction and Loss of Coolant Accident in a Pressurized Water Reactor.
_______________________________________________________________________
Abstract:
This lab allowed the observation and analysis of two large transients in a Pressurized Water Reactor simulation. This is a culmination of the prior labs in this class, allowing for an unidentified scenario to be analyzed, discovering the cause of the plant transient and identifying the key points of plant response to the two different scenarios. This lab allows for a significant amount of data to be interpreted and understood, showing an advanced understanding of integrated plant operations for nuclear power. __________________________________________________________________________
Introduction:
The primary objective of this lab is to complete two review scenarios on the PWR simulator. These scenarios will provide an opportunity to integrate the practices and knowledge obtained in prior labs, as well as to demonstrate a sufficient and practical understanding of integrated plant operations and response. PWRs have safety ingrained throughout their design, as well as a high margin of stability across different plant transients and casualties. Even a worst-case scenario is designed to be contained and mitigated with an automatic shutdown of key systems, and automatic initiation of protective functions. This design is critical to maintain safe and continuous operation of nuclear power plants. It is therefore important for to have the ability to recognize proper plant response to transients, as
well as the required response to significant casualties that may bring the plant into departure from design conditions, where damage and danger become possibilities. This lab will serve to identify these scenarios, and anaylze the appropriate plant response to ensure full understanding of critical systems, as well as confirm appropriate response to unintended events. _________________________________________________________________________
Methods:
Part B: a)
Load the PWR simulator with “Module 7: Review Scenario 1” selected. b)
Select “Run” on the ITS Panel to begin the simulation.
c)
Observe and record data to answer the following questions:
i.
Identify the plant event that is taking place.
ii.
Describe the response of the following plant system parameters:
1.
Turbine Generator Output
2.
Reactor thermal power
3.
Reactor Coolant System Parameters (Including temperatures, pressure, Pressurizer Pressure, and Pressurize Level)
4.
Main Steam System parameters (Including steam flow and steam pressure.
iii.
Describe the response of the following plant control systems:
1.
Rod Control System
2.
Pressurizer Pressure Control System
3.
Pressurizer Level Control System
iv.
Describe the response of the following Engineered Safety Feature signals and systems, if applicable:
1.
Reactor Protection System (Reactor Trip Signals)
2.
Engineered Safety Feature Signal – Reactor Coolant Injection Signal
3.
Engineered Safety Feature Signal – Containment Isolation Signal
4.
Engineered Safety Feature Signal – Main Steam Isolation Signal
5.
Engineered Safety Feature System – Reactor Coolant Injection System
Part C:
a)
Load the PWR simulator with “Module 7: Review Scenario 2” selected. b)
Select “Run” on the ITS Panel to begin the simulation.
c)
Observe and record data to answer the following questions:
i.
Identify the plant event that is taking place.
ii.
Describe the response of the following plant system parameters:
1.
Turbine Generator Output
2.
Reactor thermal power
3.
Reactor Coolant System Parameters (Including temperatures, pressure, Pressurizer Pressure, and Pressurize Level)
4.
Main Steam System parameters (Including steam flow and steam pressure.
iii.
Describe the response of the following plant control systems:
1.
Rod Control System
2.
Pressurizer Pressure Control System
3.
Pressurizer Level Control System
iv.
Describe the response of the following Engineered Safety Feature signals and systems, if applicable:
1.
Reactor Protection System (Reactor Trip Signals)
2.
Engineered Safety Feature Signal – Reactor Coolant Injection Signal
3.
Engineered Safety Feature Signal – Containment Isolation Signal
4.
Engineered Safety Feature Signal – Main Steam Isolation Signal
5.
Engineered Safety Feature System – Reactor Coolant Injection System
________________________________________________________________________
Results:
Your preview ends here
Eager to read complete document? Join bartleby learn and gain access to the full version
- Access to all documents
- Unlimited textbook solutions
- 24/7 expert homework help
Part B:
7) Utilizing data collected, answer the following questions:
i.
Identify the plant event that is taking place.
This evolution consisted of plant response to an electrical load reduction. ii.
Describe the response of the following plant system parameters:
1.
Turbine Generator Output
TG output lowered from 1400MW to 1000MW steadily as the transient occurred. The following graphic illustrates this trend. 2.
Reactor thermal power:
Reactor thermal power lowered as TG loading did, showing a normal response of following steam demand. The following graphic illustrates this trend.
3.
Reactor Coolant System Parameters (Including temperatures, pressure, Pressurizer Pressure, and Pressurize Level)
Tavg lowered slightly as the programmed Tref lowered in response
to a reduction in plant power.
Plant pressure and Pressurizer Pressure followed a matching trend, as the pressurizer is the driving control of coolant system pressure. During the initial power mismatch, there was a brief insurge into the pressurizer, causing pressure and level to rise as the steam bubble was compressed. As power lowered and coolant temperature lowered, the pressurizer went through an outsurge, causing level and pressure to lower, until the heaters activated and maintained level and pressure without significant change on the parameters. The following graphics illustrate these trends.
4.
Main Steam System parameters (Including steam flow and steam pressure.
Main Steam flow lowered throughout the transient, as the turbine throttles were shut to limit supply as load decreased. Steam Generator pressure initially rose up due to the backpressure from reduced flow, and slowly lowered back to normal values as the plant reached a steady state equilibrium. There was a brief peak in steam flow when the steam dumps opened to help normalize plant conditions. The following graphics illustrate these trends.
Your preview ends here
Eager to read complete document? Join bartleby learn and gain access to the full version
- Access to all documents
- Unlimited textbook solutions
- 24/7 expert homework help
iii.
Describe the response of the following plant control systems:
1.
Rod Control System
All rods were initially at the top of the core. The rod control system was operating with CEA Mode Select in “Auto Sequential,”
and responded to the power mismatch and Tavg-Tref mismatch by driving in the control group of rods, Group 5. At the 56 position of Group 5, Group 4 also began an insertion. As Group 4 reached the 56 position, Group 6 rods began a small insertion, securing at the 144 position. Inward motion secured for Group 5 at the 5 position bottom stop, and Group 4 at the 50 position. The following graphic
shows final rod configuration at the end of the transient. 2.
Pressurizer Pressure Control System
As pressurizer pressure initially rose, the Pressurizer Master Controller
signalled to supply spray flow into the pressurizing, slightly collapsing
the steam bubble. When the outsurge of coolant caused a decreasing pressure, the BLANK pressurizer heaters energized to maintain pressurizer pressure. As pressure was restored, the heaters deenergized.
3.
Pressurizer Level Control System
The Pressurizer Level Master Controller made continuous changes to the charging flow into the pressurizer to maintain programmed level during the insurge and outsurge. Post-transient level was slightly lower than the initial level at full power. The following graph shows pressurizer level in comparison to program level. iv.
Describe the response of the following Engineered Safety Feature signals and systems, if applicable:
1.
Reactor Protection System (Reactor Trip Signals)
There were no trip signals from the reactor protection system during this transient. 2.
Engineered Safety Feature Signal – Reactor Coolant Injection Signal
There were no Engineered Safety Features actuated during this transient. 3.
Engineered Safety Feature Signal – Containment Isolation Signal
There were no Engineered Safety Features actuated during this transient. 4.
Engineered Safety Feature Signal – Main Steam Isolation Signal
There were no Engineered Safety Features actuated during this transient. 5.
Engineered Safety Feature System – Reactor Coolant Injection System
There were no Engineered Safety Features actuated during this transient. Part C:
7) Utilizing data collected, answer the following questions:
i.
Identify the plant event that is taking place.
Rapidly lowering pressurizer level combined with a rapidly increasing containment pressure indicate a large LOCA.
ii.
Describe the response of the following plant system parameters:
6.
Turbine Generator Output
Turbine generator output dropped rapidly to 0MW when the turbine tripped. The following graphic illustrates this trend. 7.
Reactor thermal power
Reactor power went from 100% to approximately 4.5% as soon as the reactor scrammed. The 4.5% residual power is due to decay heat. The following graphic illustrates this trend. 8.
Reactor Coolant System Parameters (Including temperatures, pressure, Pressurizer Pressure, and Pressurize Level)
Reactor coolant pressure dropped substantially as the reactor tripped. Tavg quickly reached the “minimum” indication of 530,
Your preview ends here
Eager to read complete document? Join bartleby learn and gain access to the full version
- Access to all documents
- Unlimited textbook solutions
- 24/7 expert homework help
with Thot lowering down to approximately 270F, and Tcold averaging approximately 303F. As the emergency injection and cooling systems continue to operate, temperatures will continue to drive downwards. Reactor coolant pressure and pressurizer pressure followed identical trends, rapidly decreasing several hundred pounds on the initiation of the leak, and then steadily lowering down to a depressurized condition due to the size of the rupture. Pressurizer level dropped to zero almost instantly, and was the immediate major indication of the LOCA. The following graphics illustrate these trends. 9.
Main Steam System parameters (Including steam flow and steam pressure.
Steam flow dropped to zero as the steam isolation valves tripped shut. Steam pressure initially spiked due to the loss of loading, but then steadily drifted downward as the heat input from the reactor was lost. The following graphics illustrate these trends. Describe the response of the following plant control systems:
10. Rod Control System
Initially at full power, all rods were fully withdraw. In response to the reactor trip signal, all rod control devices deenergized, allowing
all rods to become fully inserted. The following graphic illustrates final rod positioning. 11. Pressurizer Pressure Control System
Upon initiation of the casualty, the pressurizer pressure master controller defaulted to a signal of 0 to prevent energization of heaters while uncovered.
12. Pressurizer Level Control System
The pressurizer level master controller attempted to make up for leakage with a high indication, driving more charging flow, but was unable to compete with the severity of the casualty and had no
effect. The following graphic illustrates the level mismatch against program band. iii.
Describe the response of the following Engineered Safety Feature signals and systems, if applicable:
13. Reactor Protection System (Reactor Trip Signals)
A large number of reactor trip signals were received throughout the
scenario due to the severity of the LOCA and its impact on various plant parameters. The initiating trip that caused the reactor to trip was PRESS PZR LOW, actuated when Pressurizer Pressure dropped below 1830psig. The following graphic shows the signaled trips shortly after the initiation of the casualty.
Your preview ends here
Eager to read complete document? Join bartleby learn and gain access to the full version
- Access to all documents
- Unlimited textbook solutions
- 24/7 expert homework help
14. Engineered Safety Feature Signal – Reactor Coolant Injection Signal
The Safety Injection Signal activated at the same time as the reactor trip, due to low pressurizer pressure. 15. Engineered Safety Feature Signal – Containment Isolation Signal
The containment isolation signal was activated when containment pressure reached 3.5psig, approximately two minutes after the initiation of the event. 16. Engineered Safety Feature Signal – Main Steam Isolation Signal
The main steam isolation signal activated when containment pressure raised above 17psig. 17. Engineered Safety Fature System – Reactor Coolant Injection System
All stages of reactor coolant injection were activated. The HPCI system was continuously injecting make up water, but was unable to compensate for the extreme leak rate in the primary system.
At approximately 600psig, the accumulators were able to discharge
into the core, rapidly lowering their level to zero.
At approximately 530psig, the RHR pumps, which had been active
already, able to inject water into the primary due to pressure lowering beneath their maximum discharge head. Pressurizer level did not restore, and pressure maintained approximately 0psig long into the simulation. The following graphics illustrate the ECF active signals, as well as operating injection systems.
__________________________________________________________________________
Discussion
:
Part B:
The first scenario illustrated a large steam demand transient in a PWR. Turbine loading decreased approximately 400MW within a few minutes, causing large changes across a variety of plant parameters. It was an excellent display of the automatic rod control system, as rod positions and speeds were adjusted in order to correct the temperature mismatch at the new power level once reactor power followed steam demand, proportionally lowering. The effects of the turbine throttles repositioning were observable in the changes in steam pressure and flow as the plant took time to reach a new equilibrium.
Your preview ends here
Eager to read complete document? Join bartleby learn and gain access to the full version
- Access to all documents
- Unlimited textbook solutions
- 24/7 expert homework help
The thermal effects also translated into pressurizer level, showing a notable transient due to the insurge and outsurge caused by large temperature changes in the reactor coolant system. The pressurizer level and pressure master controllers worked flawlessly to signal heaters, spray, and charging flowrate to compensate for these changes. Part C:
In the second simulation, a severe LOCA was initiated, causing an almost immediate trip of the reactor plant. Pressurizer level dropped out of sight almost instantly, and plant pressure rapidly decreased to a depressurized condition shortly after the initiation of the event. Although ESF systems were quick to respond and actuated correctly, they were unable to restore level or pressure within the timeframe of the simulation. Continuously lowering primary temperatures and a stable level of decay heat showed that emergency systems were successfully in providing constant decay heat removal and shutdown cooling, preventing a serious thermal event in the fuel. The containment building was successful in preventing the release of any contaminants to the surrounding environment, and the containment spray system was able to maintain pressures and temperatures within limits. The Reactor Protection Complex activated without hesitation, detecting a large series of limit violations and unsafe conditions simultaneously as well as throughout the duration of the event. This provided for immediate automatic protective features, such as the reactor trip and setting containment, which prevented this serious LOCA from escalating into a further disaster. __________________________________________________________________________
Conclusion:
This lab allowed for two important observations of reactor plant transients. The first showed a major, though well within design limits, load reduction, causing a transient that had widespread effects throughout plant systems. Rod control and pressurizer controllers played a critical role in maintaining appropriate plant parameters. The analysis of transients in pressure, temperature, and
steam pressures allowed for a detailed understanding of the response of the integrated plant systems in response to a large change in power.
The second situation was a severe LOCA, resulting in a depressurized reactor coolant system being maintained by emergency injection. This casualty showed the results of a major loss of reactor coolant inventory, and how it impacts pressure and temperature within the core. The response of the Reactor Protection complex and Engineered Safety Systems was invaluable in understanding core response to a worst-case scenario, allowing for insight and knowledge on how to adequately prepare for and respond to such events.
This lab also allowed for prior knowledge and experiences in the course to be leveraged in order to fully understand and identify the events taking place. __________________________________________________________________________
References:
3KEYSTUDENT
Generic PWR Simulator
. (n.d.). WS-Corp. https://www.3keystudent.com/
M7.5 Lab: PWR Simulations.
Excelsior University.
https://excelsior.instructure.com/courses/36956/assignments/1036158?module_item_id=3316253
Related Documents
Related Questions
Help!!! Answer it correctly!!! Make sure you solve work on hand!!! Answer correctly Please!!!!!!!
arrow_forward
a. Currently, you serve as a robotic engineer by a company, and your boss want you
to design a robot in spatial space to satisfy the following conditions:
i. Max reachable distance of the robot is 1 meter.
ii.
Only using revolute joints, connecting links and rigid load bearing links
(the length of link is 0.5m).
iii. The degree of freedom (Dof) of the robot is 2.
What is your design, how many joints and links will you use?
b. The payload of your design is 10 kg (each load bearing link can hold 5 kg, 5 kg+5
kg=10kg, the weight and payload of the connecting links will be ignored), but
your boss wants your designed robots to handle 20 kg payload. How will you
update your current design, and how many joints and links will you use in the
new design? What is the Dof of the new designed robot.
arrow_forward
Help!!! Answer all parts correctly!! Please
arrow_forward
Statics Problem !!!
Help me Part A , Part B , Part C!!!! Answer it this Problem Correctly!! Please give correct Solution
arrow_forward
!
Required information
NOTE: This is a multi-part question. Once an answer is submitted, you will be unable to return to this part.
At an intersection car B was traveling south and car A was traveling 30° north of east when they slammed into each other.
Upon investigation, it was found that after the crash, the two cars got stuck and skidded off at an angle of 10° north of east.
Each driver claimed that he was going at the speed limit of 50 km/h and that he tried to slow down, but couldn't avoid the
crash because the other driver was going a lot faster. The masses of cars A and B were 1500 kg and 1200 kg, respectively.
10°
30°
VR
Determine the speed of the faster of the two cars if the slower car was traveling at the speed limit.
The speed of the faster car was km/h.
arrow_forward
Help with this would be great, thanks!
arrow_forward
Help with this would be great, thanks!
arrow_forward
I just need help with part D, thanks
arrow_forward
Help!!! Please answer part A and part B correctly!!! Please!!!
arrow_forward
MENG364 MANUFACTURING TECHNOLOGY
please solve the question very quickly
Student Number 124544
arrow_forward
I want to answer all the questions by handwriting.
arrow_forward
Do not give answer in image and hand writing
arrow_forward
answer a & b. Please show all your work!!
arrow_forward
I asked for problems 6 and 7 to be answered, but I did not get a properly structured answered as the example shows on problem number 1. Here is the link to the questions I already had answered, could you please rewrite the answer so its properly answered as the example shows (Problem 1)?
https://www.bartleby.com/questions-and-answers/it-vivch-print-reading-for-industry-228-class-date-name-review-activity-112-for-each-local-note-or-c/cadc3f7b-2c2f-4471-842b-5a84bf505857
arrow_forward
Explanation it correctly not use excel
Q)land development company is considering the purchase of earth-moving equipment. This equipment will have an estimated first cost of $163,000, a salvage value of $65,000, a life of 10 years, a maintenance cost of $40,000 per year, and an operating cost of $420 per day. Alternatively, the company can rent the necessary equipment for $1030 per day and hire a driver at $180 per day.
If the company’s MARR is 15% per year, how many days per year must the company need the equipment in order to justify its purchase?
The company must need the equipment ____ days per year in order to justify its purchase.
arrow_forward
Are you as strong as a horse?
Purpose: To understand the concept of work and power and to calculate the power
generated by yourself or a friend.
Materials: A tape measure, stairs, and a stopwatch, cell phone, or wristwatch.
Key Physics Concept: Power is the rate of doing work. Power can be calculated by
dividing the work done by the time it takes to do the work; in this case we will do work
against gravity on a stair case (you may add your power in doing pushups to test your
arm power). Can you guess what your peak horsepower is?
You may do the stairs one at
at time or multiple stairs at a
a time; whatever is most safe
and efficient for you.
Procedure:
Observations:
Analysis:
Conclusions:
Application:
1. Will your hp change if you performed the activity for a longer duration (for example,
climbed the CN tower staircase)? In what way? Explain.
2. Research the horsepower generated by a car. How does the average person's peak
power rating compare to it? How many average people would it…
arrow_forward
I Review
Learning Goal:
To be able to find the center of gravity, the center of
mass, and the centroid of a composite body.
Part A
A centroid is an object's geometric center. For an object
of uniform composition, its centroid is also its center of
mass. Often the centroid of a complex composite body is
found by, first, cutting the bodyng the weighted average
Find the area of the body. (Figure 1)
Express your answer numerically in feet squared to three significant figures.
into regular shaped
segments, and then by calculating i
of the segments' centroids.An object is made from a
uniform piece of sheet metal. The object has dimensions
of a = 1.55 ft , where a is the diameter of the semi-
circle,b = 3.74 ft, and c = 2.35 ft . A hole with diameter
d = 0.750 ft is centered at (1.26, 0.775).
> View Available Hint(s)
Vol AEO It vec
?
A =
ft?
Submit
Part B
Find a, the a-coordinate of the body's centroid. (Figure 1)
Express your answer numerically in feet to three significant figures.
> View…
arrow_forward
Problem 3: A small gearing system in your brand-new 3D printer fails as you were printing,
leading to an overload in the motor and a fire in your home. The fire spreads, burning half of
Everglades National Park in the worst environmental disaster in US history.
The printer company blames you! Stating that you must have been misusing the printer some-
how. Use your engineering knowledge to "show those jerks you mean business!"
You investigate and find a serial number on the gears and type it into Google, finding the
company that manufactures the gears. Their website provides some info that this series of gear is
made from AISI 4340 steel and the yield strength is 125 kpsi.
Assuming each gear tooth acts as a small cantilever, prove to the 3D printer company that they
are liable for the fire.
oct
#
Gear Information
Gear radius (r): 1.00 in
Tooth length (1): 0.15 in
Tooth height (h): 0.15 in
Tooth thickness (b): 0.1 in
Angular velocity (w): 0.1 rad/s
Motor Information
Power (H): 0.009 hp
1…
arrow_forward
Solve all parts
arrow_forward
Dynamics
arrow_forward
Mech. Engg. Dept.
4th year 2022-2023
Solar Energy
Spring course MEC364
Dr. Mahmoud U. Jasim
Review/Recap Sheet
Q1- Answer with true or false and rewrite the false statements completely in
correct form, otherwise no mark will be put on the false statements.
1
2
To represent a location on earth surface you need to define its altitude and longitude
angles.
3
Solar zenith and solar incidence angles have the same value for horizontal surface.
At sunset time the value of solar altitude angle is maximum.
4
The angle which represents the inclination of a given surface is the zenith angle
5
6
7
8
When the absolute value of sun-wall azimuth angle exceeds 90' this means that the sun
rays are reaching the receiving plane.
The solar irradiance and the solar irradiation have the same physical meaning.
In the case of clear sky weather, the beam solar irradiation on a horizontal surface is less
than the diffused irradiation.
The total solar radiation received by a tilted surface is the same as that…
arrow_forward
Can tutor explain clearly and step by step by explanation on each questions
a)Calculate the Gear Ratio.
b)Calculate the helicopter rotor blade’s rotational speed.
c)Calculate the lift generated by the helicopter rotor blade.
d)Calculate the force required for circular motion.
e)Calculate the forward velocity of the helicopter.
f)Calculate the time to complete one circular motion.
g)Is there vibration experienced by the helicopter?
i.If yes, what caused it and how to reduce this vibration?
h)How to double the time for (f)?
arrow_forward
SEE MORE QUESTIONS
Recommended textbooks for you
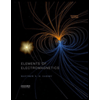
Elements Of Electromagnetics
Mechanical Engineering
ISBN:9780190698614
Author:Sadiku, Matthew N. O.
Publisher:Oxford University Press
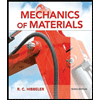
Mechanics of Materials (10th Edition)
Mechanical Engineering
ISBN:9780134319650
Author:Russell C. Hibbeler
Publisher:PEARSON
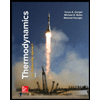
Thermodynamics: An Engineering Approach
Mechanical Engineering
ISBN:9781259822674
Author:Yunus A. Cengel Dr., Michael A. Boles
Publisher:McGraw-Hill Education
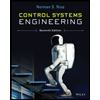
Control Systems Engineering
Mechanical Engineering
ISBN:9781118170519
Author:Norman S. Nise
Publisher:WILEY
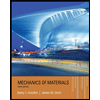
Mechanics of Materials (MindTap Course List)
Mechanical Engineering
ISBN:9781337093347
Author:Barry J. Goodno, James M. Gere
Publisher:Cengage Learning
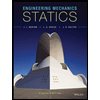
Engineering Mechanics: Statics
Mechanical Engineering
ISBN:9781118807330
Author:James L. Meriam, L. G. Kraige, J. N. Bolton
Publisher:WILEY
Related Questions
- Help!!! Answer it correctly!!! Make sure you solve work on hand!!! Answer correctly Please!!!!!!!arrow_forwarda. Currently, you serve as a robotic engineer by a company, and your boss want you to design a robot in spatial space to satisfy the following conditions: i. Max reachable distance of the robot is 1 meter. ii. Only using revolute joints, connecting links and rigid load bearing links (the length of link is 0.5m). iii. The degree of freedom (Dof) of the robot is 2. What is your design, how many joints and links will you use? b. The payload of your design is 10 kg (each load bearing link can hold 5 kg, 5 kg+5 kg=10kg, the weight and payload of the connecting links will be ignored), but your boss wants your designed robots to handle 20 kg payload. How will you update your current design, and how many joints and links will you use in the new design? What is the Dof of the new designed robot.arrow_forwardHelp!!! Answer all parts correctly!! Pleasearrow_forward
- Statics Problem !!! Help me Part A , Part B , Part C!!!! Answer it this Problem Correctly!! Please give correct Solutionarrow_forward! Required information NOTE: This is a multi-part question. Once an answer is submitted, you will be unable to return to this part. At an intersection car B was traveling south and car A was traveling 30° north of east when they slammed into each other. Upon investigation, it was found that after the crash, the two cars got stuck and skidded off at an angle of 10° north of east. Each driver claimed that he was going at the speed limit of 50 km/h and that he tried to slow down, but couldn't avoid the crash because the other driver was going a lot faster. The masses of cars A and B were 1500 kg and 1200 kg, respectively. 10° 30° VR Determine the speed of the faster of the two cars if the slower car was traveling at the speed limit. The speed of the faster car was km/h.arrow_forwardHelp with this would be great, thanks!arrow_forward
arrow_back_ios
SEE MORE QUESTIONS
arrow_forward_ios
Recommended textbooks for you
- Elements Of ElectromagneticsMechanical EngineeringISBN:9780190698614Author:Sadiku, Matthew N. O.Publisher:Oxford University PressMechanics of Materials (10th Edition)Mechanical EngineeringISBN:9780134319650Author:Russell C. HibbelerPublisher:PEARSONThermodynamics: An Engineering ApproachMechanical EngineeringISBN:9781259822674Author:Yunus A. Cengel Dr., Michael A. BolesPublisher:McGraw-Hill Education
- Control Systems EngineeringMechanical EngineeringISBN:9781118170519Author:Norman S. NisePublisher:WILEYMechanics of Materials (MindTap Course List)Mechanical EngineeringISBN:9781337093347Author:Barry J. Goodno, James M. GerePublisher:Cengage LearningEngineering Mechanics: StaticsMechanical EngineeringISBN:9781118807330Author:James L. Meriam, L. G. Kraige, J. N. BoltonPublisher:WILEY
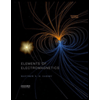
Elements Of Electromagnetics
Mechanical Engineering
ISBN:9780190698614
Author:Sadiku, Matthew N. O.
Publisher:Oxford University Press
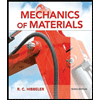
Mechanics of Materials (10th Edition)
Mechanical Engineering
ISBN:9780134319650
Author:Russell C. Hibbeler
Publisher:PEARSON
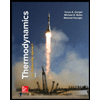
Thermodynamics: An Engineering Approach
Mechanical Engineering
ISBN:9781259822674
Author:Yunus A. Cengel Dr., Michael A. Boles
Publisher:McGraw-Hill Education
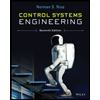
Control Systems Engineering
Mechanical Engineering
ISBN:9781118170519
Author:Norman S. Nise
Publisher:WILEY
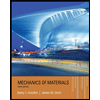
Mechanics of Materials (MindTap Course List)
Mechanical Engineering
ISBN:9781337093347
Author:Barry J. Goodno, James M. Gere
Publisher:Cengage Learning
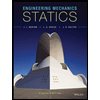
Engineering Mechanics: Statics
Mechanical Engineering
ISBN:9781118807330
Author:James L. Meriam, L. G. Kraige, J. N. Bolton
Publisher:WILEY