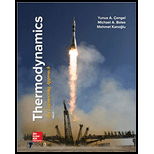
a)
The rate of exergy destroyed during the process and the exit temperature
a)

Answer to Problem 66P
The rate of exergy destroyed during the process is
The exit temperature
Explanation of Solution
Draw the schematic diagram of the flow of refrigerant-134a through evaporator section as shown in Figure (1).
Write the expression for the mass balances equation for the heat exchanger.
Here, mass flow rate of refrigerant at inlet is
Since net mass flow rate of refrigerant-134a and air through system is 0.
From Figure (1), the mass flow rate of refrigerant-134a at
Here, initial and final mass flow rate of refrigerant at
From Figure (1), the mass flow rate of air at
Here, mass flow rate of air at
Write the expression for the enthalpy at state 1
Write the expression for the entropy at state 1
Write the expression for the mass flow rate of air
Here, gas constant of air is
Write the expression for energy balance for the heat exchanger
Here, rate of net energy transfer in to the control volume is
Substitute 0 for
Here, mass flow rate at
Write the expression for the entropy balance for the steady flow system as;
Here, rate of entropy generation is
At steady state, rate of change in entropy of the system is zero.
Substitute 0 for
Here, entropy at
Write the expression for the change between state 4 entropy
Here, temperature at state
Write the expression for the exergy destroyed rate during the process
Here, dead state temperature is
Conclusion:
Refer to Table A-12, “Saturated refrigerant-134a-Pressure table”, obtain the following properties at the pressure
Here, enthalpy of saturated liquid is
Substitute
Substitute
Refer to Table A-12, “Saturated refrigerant-134a-Pressure table”, obtain the following properties at the pressure
Here, enthalpy at state 2 is
From the Table A-2, “Ideal-gas specific heats of various common gases table”, select the gas constant of air gas
Substitute
At steady state, rate of change in internal energy of the system is zero.
From the Table A-2, “Ideal-gas specific heats of various common gases table”, select the constant pressure specific heat
Substitute
Thus, the exit temperature
Substitute
Substitute
Substitute
Thus, the rate of exergy destroyed during the process is
b)
The exit temperature of the air and the rate of exergy destroyed during the process without insulation.
b)

Answer to Problem 66P
The exit temperature of the air without insulation is
The rate of exergy destroyed during the process without insulation is
Explanation of Solution
Write the expression for the state 4 temperature
Here, heat gain is from the surrounding
Write the expression for the entropy balance For an extended system as;
Conclusion:
Substitute
Thus, the exit temperature of the air is
substitute
Substitute
Substitute
Thus, the rate of exergy destroyed during the process is
Want to see more full solutions like this?
Chapter 8 Solutions
THERMODYNAMICS (LL)-W/ACCESS >CUSTOM<
- A beam supports a uniform load and an axial load P = 30 kips. If the maximum allowable tensile stress in the beam is 24 ksi and a maximum allowable compressive stress is 20 ksi, what uniform load can the beam support? Assume P passes through the centroid of the section.arrow_forwardBending Moment Value? 40 kN 100 kN 100 kN 100 kN 40 kN A B C D E Ym Zm Zm Ym X = ?arrow_forward(4) Figure Q4 shows a symmetrically loaded beam. The beam is loaded at position A (x = 0 m) and the end of the beam at position E with 30 kN. There is an additional load of 101 kN both at position B (Y = 0.87 m), in the middle at C and at position D. The middle section is 2Z, where Z = 0.82 m). Given that the reaction forces at RB and RD both equal 180 kN, calculate the Bending Moment value (using the convention given to you in the module's formula book) at a position of x=2.30m. State your answer in terms of kilo-Newton-metres to one decimal place. Bending Moment Value? 40 kN 100 kN 100 kN 100 kN 40 kN B D E Ym Zm Zm Ym X = ? Figure Q4arrow_forward
- (8) Figure Q8 shows a T cross-section of a T beam which is constructed from three metal plates each having a width of 12 mm and sectional engths of X=72 mm, Y=65 mm and Z=88 mm, where the plates are used for the web section, and the two flange sections respectively, as llustrated in Figure Q8. Calculate the neutral axis of the T-beam cross-section (as measured from the base) in units of millimetres, stating your answer to the nearest 1 decimal place. Z mm Y mm 12 mm X mm Figure Q8 12 mm 12 mmarrow_forward(10) A regular cross-section XXY mm beam, where X-94 m and Y=62 m and 1800 mm long, is loaded from above in the middle with a load of Z=2 kN causing a compressive Bending Stress at the top of the beam and tensile Bending Stress at the bottom of the beam. The beam in addition experiences a tensile end loading in order to reduce the compressive stress in the beam to a near zero value. The configuration of the beam is illustrated in Figure Q10. Calculate the end loading force required in order to reduce total compressive stress experienced in the beam to be near zero? State your answer to the nearest 1 decimal place in terms of kilo-Newtons. Z kN Y mm 1800 mm X mm ? KN Figure Q10 ? KNarrow_forward(12) Figure Q12 shows a framework consisting of 3 upward pointing isosceles triangles and 2 downward pointing isosceles triangles. The framework is loaded at joint F with a downward force of 20 kN. The applied force causes a vertical reaction force at A and D. The design of the framework is such that horizontal base of the isosceles triangles form an angle of 30° degrees with the diagonal members. You are asked to find the internal force in member AE in kilo-Newtons to 1 decimal place (using the standard sign convention given in the module formula booklet)? Select the valid option from the list below. E F S 20 kN RAX = ?? KN 30° 30° 30° 30° 30° 30° A H H B D RAV = ?? KN Roy = ?? KN A. The solution to the problem is found to be -20.0 kN. ○ B. The solution to the problem is found to be -10.0 kN. ○ C. The solution to the problem is found to be +11.5 kN. OD. The solution to the problem is found to be +23.1 kN. O E. No Valid Answerarrow_forward
- (14) An inverted T beam is constructed from a top square cross-section section and a bottom rectangular cross-section of the same length. The cross-section dimensions of the sections are as follows: - Top Square Section 30 mm x 30 mm (width x depth) Bottom Rectangular Section 50 mm x 30 mm Figure Q14 shows the cross-section arrangement of the plates. Given that compression and tension behave the same in terms of stress analysis. Calculate the distance, Ymax, you would use to calculate a safe bending stress value for further analysis. You are required to state your answer in millimetres to the nearest whole number. 30 mm 30 mm O O A. 34 B. 26 O c. 33 D.27 ○ E. No Valid Answer 30 mm 50 mm Figure Q14 1marrow_forward(15) A block of metal with a Young's Modulus of E=200 GPa and Poisson's ratio of 0.3, has dimensions of 38 mm × 20 mm x 80 mm for the lengths X, Y and Z respectively as illustrated in Figure Q15. The block experiences a tensile force in the x-direction of 100 kN and also an applied tensile force in the z-direction of 200 kN as illustrated in Figure Q15. Calculate the strain experienced in the x-direction in terms of micro-strain. Stating your answer to the nearest whole number. 100 kN 200 kN X=38 mm Y = 20 mm ○ A.-188 microstrain OB. -82 microstrain ○ c. no valid answer OD. +83 microstrain ○ E. -187 microstrain Z Figure Q15 200 kN Z = 80 mm 100 kN y Xarrow_forwardFigure Q3 shows a symmetrically loaded beam, loaded with a single Uniform Distributed Load (UDL) starting from the leftmost position A (x = 0 m) ending at the end of the beam at the rightmost position D. The UDL has loading case of 10 kN/m, see Figure Q3 for the start and end positions. There are two symmetrically located pivots causing reaction forces of RB at position B (Y = 1.3 m) and RC at position C. The central section of the beam spans for 2.4 m. Calculate the Shear Force value at a position of X=1.9 m. State your answer in kilo-Newtons to one decimal place.arrow_forward
- (6) An I beam that is Z=685 mm long has a symmetric cross-section shown in Figure Q6. The lower and upper sections are 2Y wide and the middle section of the I beam is Y wide, where Y=44 mm wide. All three sections have a depth of 44mm, as illustrated in Figure Q6. The I beam is pulled apart by a force of X=32 kN. What is the maximum stress experienced in the shaft in terms of mega-Pascals. State your answer to 1 decimal place. Y mm F = X KN Y mm Y mm Y mm 2Y mm Z mm Figure Q6 F= X KNarrow_forward(7) A solid shaft of diameter X=18 mm and length of Y=1.4 m experiences torque using a short rod that is Z=520 mm long and is fixed at the open end of shaft experiencing the torque. The torque is created with the application of a 760 N perpendicular force. The set-up is illustrated below in Figure Q7. Given the shaft has a shear modulus of 70 GPa, calculate the angle of twist in terms of degrees? State your answer to the nearest whole number. Ym Figure Q7 X mm 750 NA Z mmarrow_forwardCalculate the strain experienced in the x-direction in terms of micro-strain. Stating your answer to the nearest whole number. 100 kN 200 kN X=38 mm A. +83 microstrain B. no valid answer ○ C.-187 microstrain ○ D.-82 microstrain OE. -188 microstrain Y = 20 mm Z Figure Q15 200 kN Z = 80 mm 100 kN y Xarrow_forward
- Elements Of ElectromagneticsMechanical EngineeringISBN:9780190698614Author:Sadiku, Matthew N. O.Publisher:Oxford University PressMechanics of Materials (10th Edition)Mechanical EngineeringISBN:9780134319650Author:Russell C. HibbelerPublisher:PEARSONThermodynamics: An Engineering ApproachMechanical EngineeringISBN:9781259822674Author:Yunus A. Cengel Dr., Michael A. BolesPublisher:McGraw-Hill Education
- Control Systems EngineeringMechanical EngineeringISBN:9781118170519Author:Norman S. NisePublisher:WILEYMechanics of Materials (MindTap Course List)Mechanical EngineeringISBN:9781337093347Author:Barry J. Goodno, James M. GerePublisher:Cengage LearningEngineering Mechanics: StaticsMechanical EngineeringISBN:9781118807330Author:James L. Meriam, L. G. Kraige, J. N. BoltonPublisher:WILEY
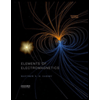
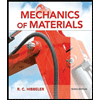
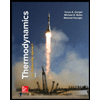
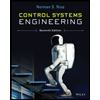
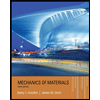
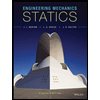