PRINT COMPANION ENGINEER THERMO
9th Edition
ISBN: 9781119778011
Author: MORAN
Publisher: WILEY
expand_more
expand_more
format_list_bulleted
Question
Chapter 4, Problem 4.61P
a.
To determine
The mass flow rate at the exit.
b.
To determine
The specific enthalpy at exit.
c.
To determine
The temperature in
Expert Solution & Answer

Want to see the full answer?
Check out a sample textbook solution
Students have asked these similar questions
As shown in the figure, Refrigerant 22 enters the compressor of an air conditioning unit operating at steady state at 40oF, 80 lbf/in2 and is compressed to 160oF, 200 lbf/in2. The refrigerant exiting the compressor enters a condenser where energy transfer to air as a separate stream occurs, and the refrigerant exits as a liquid at 200 lbf/in2, 90oF. Air enters the condenser at 75oF, 14.7 lbf/in2 with a volumetric flow rate of 1500 ft3/min and exits at 110oF. Neglect stray heat transfer and kinetic and potential energy effects, and assume ideal gas behavior for the air.
As shown in the figure, Refrigerant 22 enters the compressor of an air conditioning unit operating at steady state at 40oF, 80 lbf/in2 and is compressed to 160oF, 200 lbf/in2. The refrigerant exiting the compressor enters a condenser where energy transfer to air as a separate stream occurs, and the refrigerant exits as a liquid at 200 lbf/in2, 90oF. Air enters the condenser at 70oF, 14.7 lbf/in2 with a volumetric flow rate of 1500 ft3/min and exits at 110oF. Neglect stray heat transfer and kinetic and potential energy effects, and assume ideal gas behavior for the air.
Current Attempt in Progress
A pump is used to circulate hot water in a home heating system. Water enters the well-insulated pump operating at steady state at a
rate of 0.42 gal/min. The inlet pressure and temperature are 14.7 Ibf/in.?, and 180°F, respectively; at the exit the pressure is 90 Ibf/in.2
The pump requires 1/25 hp of power input. Water can be modeled as an incompressible substance with constant density of 60.58
Ib/ft and constant specific heat of 1 Btu/lb · °R.
Neglecting kinetic and potential energy effects, determine the temperature change, in °R, as the water flows through the pump.
AT =
i
°R
Chapter 4 Solutions
PRINT COMPANION ENGINEER THERMO
Ch. 4 - Prob. 4.1ECh. 4 - Prob. 4.2ECh. 4 - Prob. 4.3ECh. 4 - Prob. 4.4ECh. 4 - Prob. 4.5ECh. 4 - Prob. 4.6ECh. 4 - Prob. 4.7ECh. 4 - Prob. 4.8ECh. 4 - Prob. 4.9ECh. 4 - Prob. 4.10E
Ch. 4 - Prob. 4.11ECh. 4 - Prob. 4.12ECh. 4 - Prob. 4.13ECh. 4 - Prob. 4.14ECh. 4 - Prob. 4.15ECh. 4 - Prob. 4.1CUCh. 4 - Prob. 4.2CUCh. 4 - Prob. 4.3CUCh. 4 - Prob. 4.4CUCh. 4 - Prob. 4.5CUCh. 4 - Prob. 4.6CUCh. 4 - Prob. 4.7CUCh. 4 - Prob. 4.8CUCh. 4 - Prob. 4.9CUCh. 4 - Prob. 4.10CUCh. 4 - Prob. 4.11CUCh. 4 - Prob. 4.12CUCh. 4 - Prob. 4.13CUCh. 4 - Prob. 4.14CUCh. 4 - Prob. 4.15CUCh. 4 - Prob. 4.16CUCh. 4 - Prob. 4.17CUCh. 4 - Prob. 4.18CUCh. 4 - Prob. 4.19CUCh. 4 - Prob. 4.20CUCh. 4 - Prob. 4.21CUCh. 4 - Prob. 4.22CUCh. 4 - Prob. 4.23CUCh. 4 - Prob. 4.24CUCh. 4 - Prob. 4.25CUCh. 4 - Prob. 4.26CUCh. 4 - Prob. 4.27CUCh. 4 - Prob. 4.28CUCh. 4 - Prob. 4.29CUCh. 4 - Prob. 4.30CUCh. 4 - Prob. 4.31CUCh. 4 - Prob. 4.32CUCh. 4 - Prob. 4.33CUCh. 4 - Prob. 4.34CUCh. 4 - Prob. 4.35CUCh. 4 - Prob. 4.36CUCh. 4 - Prob. 4.37CUCh. 4 - Prob. 4.38CUCh. 4 - Prob. 4.39CUCh. 4 - Prob. 4.40CUCh. 4 - Prob. 4.41CUCh. 4 - Prob. 4.42CUCh. 4 - Prob. 4.43CUCh. 4 - Prob. 4.44CUCh. 4 - Prob. 4.45CUCh. 4 - Prob. 4.46CUCh. 4 - Prob. 4.47CUCh. 4 - Prob. 4.48CUCh. 4 - Prob. 4.49CUCh. 4 - Prob. 4.50CUCh. 4 - Prob. 4.51CUCh. 4 - Prob. 4.1PCh. 4 - Prob. 4.2PCh. 4 - Prob. 4.3PCh. 4 - Prob. 4.4PCh. 4 - Prob. 4.5PCh. 4 - Prob. 4.6PCh. 4 - Prob. 4.7PCh. 4 - Prob. 4.8PCh. 4 - Prob. 4.9PCh. 4 - Prob. 4.10PCh. 4 - Prob. 4.11PCh. 4 - Prob. 4.12PCh. 4 - Prob. 4.13PCh. 4 - Prob. 4.14PCh. 4 - Prob. 4.15PCh. 4 - Prob. 4.16PCh. 4 - Prob. 4.17PCh. 4 - Prob. 4.18PCh. 4 - Prob. 4.19PCh. 4 - Prob. 4.20PCh. 4 - Prob. 4.21PCh. 4 - Prob. 4.22PCh. 4 - Prob. 4.23PCh. 4 - Prob. 4.24PCh. 4 - Prob. 4.25PCh. 4 - Prob. 4.26PCh. 4 - Prob. 4.27PCh. 4 - Prob. 4.28PCh. 4 - Prob. 4.29PCh. 4 - Prob. 4.30PCh. 4 - Prob. 4.31PCh. 4 - Prob. 4.32PCh. 4 - Prob. 4.33PCh. 4 - Prob. 4.34PCh. 4 - Prob. 4.35PCh. 4 - Prob. 4.36PCh. 4 - Prob. 4.37PCh. 4 - Prob. 4.38PCh. 4 - Prob. 4.39PCh. 4 - Prob. 4.40PCh. 4 - Prob. 4.41PCh. 4 - Prob. 4.42PCh. 4 - Prob. 4.43PCh. 4 - Prob. 4.44PCh. 4 - Prob. 4.45PCh. 4 - Prob. 4.46PCh. 4 - Prob. 4.47PCh. 4 - Prob. 4.48PCh. 4 - Prob. 4.49PCh. 4 - Prob. 4.50PCh. 4 - Prob. 4.51PCh. 4 - Prob. 4.52PCh. 4 - Prob. 4.53PCh. 4 - Prob. 4.54PCh. 4 - Prob. 4.55PCh. 4 - Prob. 4.56PCh. 4 - Prob. 4.57PCh. 4 - Prob. 4.58PCh. 4 - Prob. 4.59PCh. 4 - Prob. 4.60PCh. 4 - Prob. 4.61PCh. 4 - Prob. 4.62PCh. 4 - Prob. 4.63PCh. 4 - Prob. 4.64PCh. 4 - Prob. 4.65PCh. 4 - Prob. 4.66PCh. 4 - Prob. 4.67PCh. 4 - Prob. 4.68PCh. 4 - Prob. 4.69PCh. 4 - Prob. 4.70PCh. 4 - Prob. 4.71PCh. 4 - Prob. 4.72PCh. 4 - Prob. 4.73PCh. 4 - Prob. 4.74PCh. 4 - Prob. 4.75PCh. 4 - Prob. 4.76PCh. 4 - Prob. 4.77PCh. 4 - Prob. 4.78PCh. 4 - Prob. 4.79PCh. 4 - Prob. 4.80PCh. 4 - Prob. 4.81PCh. 4 - Prob. 4.82PCh. 4 - Prob. 4.83PCh. 4 - Prob. 4.84PCh. 4 - Prob. 4.85PCh. 4 - Prob. 4.86PCh. 4 - Prob. 4.87PCh. 4 - Prob. 4.88P
Knowledge Booster
Learn more about
Need a deep-dive on the concept behind this application? Look no further. Learn more about this topic, mechanical-engineering and related others by exploring similar questions and additional content below.Similar questions
- Thank youarrow_forwardT-7arrow_forwardA well-insulated turbine operating at steady state, produces 23 MW of power with a steam flow rate of 40 kg/h. Water vapor enters the turbine at 360°C at a speed of 35 m/s. It comes out from turbine at 0.06 bar pressure and 120 m/s velocity as saturated steam. Potential energy effects are neglected. Determine the inlet pressure in bar.arrow_forward
- Problem 5.060 SI The figure shows the schematic of a vapor power plant in which 100 kg/s of water circulates through the four components operating at steady state. The water flows through the boiler and condenser at constant pressure and through the turbine and pump adiabatically. Kinetic and potential energy effects can be ignored. Process data follow: Process 4-1: constant-pressure at 4000 kPa from saturated liquid to saturated vapor. Process 2-3: constant-pressure at 20 kPa from x2 = 88% to x3 = 18%. m = 100 kg/s Boiler X2 = 88% X3 = 18% Water Pump Turbine Condenser 2 Determine thermal efficiency. Determine ởrvle, in kW/K. Determine if the cycle is internally reversible, irreversible, or impossible. v Step 1 Determine the cycle thermal efficiency. % the tolerance is +/-2% Click if you would like to Show Work for this question: Open Show Work By accessing this Question Assistance, you will learn while you earn points based on the Point Potential Policy set by your instructor.…arrow_forwardAt steady state, Refrigerant 22 enters (1) the compressor at 40C, 5.5bar and is compressed to 60C, 13.8bar. R-22 exiting (2) the compressor enters a heat exchanger where energy transfer to air as a separate stream occurs and the refrigerant exits (3) as a liquid at 13.5bar, 32C. Air enters (4) the condenser at 27C, 1.0bar with a volumetric flow rate of 21.2m3/min and exits (5) at 43C. Assuming ideal gas behavior for the air and stray heat transfer and kinetic and potential energy effects are negligible, determine the compressor powerarrow_forwardSteam at 44 bar and a dryness fraction, x = 0.9 is throttled to a pressure of 12 bar. Calculate thedifference in power output in kilowatts between the following two expansion processes:a) Steam at the initial pressure of 44 bar and x = 0.9 at State 1 is expanded in a turbine to State 3 at 0.12 bar.b) Steam at the reduced pressure of 12 bar after throttling at State 2 is expanded in another turbine to State 4 at the same exhaust pressure of 0.12 bar.The mass flow rate of steam is 8 kg/sec in both cases and the expansion in both turbines can be assumed to be reversible and adiabatic. Sketch both expansion processes on the same T-s diagram using the respective initial and final state points as described above.Explain the reason for the difference in power output.Calculate the mass flow rate of steam for the turbine operating at the throttled/reduced pressure to generate the same output as the turbine operating at the pressure before throttling.NOTE: You are required to number the state…arrow_forward
- Thanksarrow_forwardA pump is used to circulate hot water in a home heating system. Water enters the well-insulated pump operating at steady state at a rate of 0.42 gal/min. The inlet pressure and temperature are 14.7 lbf/in.2, and 180°F, respectively; at the exit the pressure is 120 lbf/in.2 The pump requires 1/25 hp of power input. Water can be modeled as an incompressible substance with constant density of 60.58 lb/ft3 and constant specific heat of 1 Btu/lb · °R.Neglecting kinetic and potential energy effects, determine the temperature change, in °R, as the water flows through the pump.arrow_forwardA pump is used to circulate hot water in a home heating system. Water enters the well-insulated pump operating at steady state at a rate of 0.42 gal/min. The inlet pressure and temperature are 14.7 lbf/in.2, and 180°F, respectively; at the exit the pressure is 60 lbf/in.2 The pump requires 1/ 35 hp of power input. Water can be modeled as an incompressible substance with constant density of 60.58 lb/ft3 and constant specific heat of 1 Btu/lb · °R. Neglecting kinetic and potential energy effects, determine the temperature change, in °R, as the water flows through the pump.arrow_forward
- A feed water heater operating in steady state has 2 inlets and 1 outlet. Liquid water enters. from the 1st inlet with 7 bar pressure, 42°C temperature and 70 kg/s mass flow.From the inlet 2, a liquid-steam mixture enters to the system with 98% quality and 7 bar pressure. Saturated liquid comes out from the outlet at 7 bar pressure.Heat transfer with the environment, kinetic and potential energy effects are neglected. Find the mass flow entering the 2nd inlet. Find the mass flow entering from the 2nd inlet. (kg/s)arrow_forwardAs shown in the figure, Refrigerant 22 enters the compressor of an air conditioning unit operating at steady state at 40°F, 80 lbp/in² and is compressed to 160°F. 200 lb/in?. The refrigerant exiting the compressor enters a condenser where energy transfer to air as a separate stream occurs, and the refrigerant exits as a liquid at 200 lb/in², 90°F. Air enters the condenser at 75°F, 14.7 lb/in² with a volumetric flow rate of 1000 ft3/min and exits at 110°F. Neglect stray heat transfer and kinetic and potential energy effects, and assume ideal gas behavior for the air. Step 1 Compresser 1+R22 Hint Step 2 mg22 = 7.026 7-11 wwwww www Wa= 7₂=160 F P-200 7₁-4FF P-80² Your answer is correct. Determine the mass flow rate of refrigerant, in lb/min. Determine the mass flow rate of refrigerant, in lb/min, and the compressor power, in horsepower. * Your answer is incorrect. 2.125 -Condemer lb/min Determine the compressor power, in horsepower. Aus at T P 1471² (AV) 7₁-90°F Py = 200 m² hp P == 300…arrow_forwardAs shown in the figure, Refrigerant 22 enters the compressor of an air conditioning unit operating at steady state at 40°F, 80 lb/in² and is compressed to 160°F, 200 lb/in². The refrigerant exiting the compressor enters a condenser where energy transfer to air as a separate stream occurs, and the refrigerant exits as a liquid at 200 lb/in², 90°F. Air enters the condenser at 70°F, 14.7 lb-/in² with a volumetric flow rate of 1500 ft³/min and exits at 110°F. Neglect stray heat transfer and kinetic and potential energy effects, and assume ideal gas behavior for the air. Step 1 I₁-110°F Compressor 1+ R22 at MR22 = www www T₂-160°F P₁-200 lbfin.² Condenser Air at T₁ P4-14.71bfin.² (AV), 7₁-90°F P-200 lbf/in² T₁=40°F Pi-80 lbfin.² Determine the mass flow rate of refrigerant, in lb/min, and the compressor power, in horsepower. Determine the mass flow rate of refrigerant, in lb/min. lb/min T₂ <- 60°F T₁ = 90°F T₁ = 40°F Pa = Pa = 200 bin² P-801brin²arrow_forward
arrow_back_ios
SEE MORE QUESTIONS
arrow_forward_ios
Recommended textbooks for you
- Elements Of ElectromagneticsMechanical EngineeringISBN:9780190698614Author:Sadiku, Matthew N. O.Publisher:Oxford University PressMechanics of Materials (10th Edition)Mechanical EngineeringISBN:9780134319650Author:Russell C. HibbelerPublisher:PEARSONThermodynamics: An Engineering ApproachMechanical EngineeringISBN:9781259822674Author:Yunus A. Cengel Dr., Michael A. BolesPublisher:McGraw-Hill Education
- Control Systems EngineeringMechanical EngineeringISBN:9781118170519Author:Norman S. NisePublisher:WILEYMechanics of Materials (MindTap Course List)Mechanical EngineeringISBN:9781337093347Author:Barry J. Goodno, James M. GerePublisher:Cengage LearningEngineering Mechanics: StaticsMechanical EngineeringISBN:9781118807330Author:James L. Meriam, L. G. Kraige, J. N. BoltonPublisher:WILEY
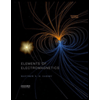
Elements Of Electromagnetics
Mechanical Engineering
ISBN:9780190698614
Author:Sadiku, Matthew N. O.
Publisher:Oxford University Press
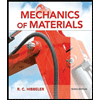
Mechanics of Materials (10th Edition)
Mechanical Engineering
ISBN:9780134319650
Author:Russell C. Hibbeler
Publisher:PEARSON
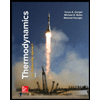
Thermodynamics: An Engineering Approach
Mechanical Engineering
ISBN:9781259822674
Author:Yunus A. Cengel Dr., Michael A. Boles
Publisher:McGraw-Hill Education
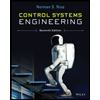
Control Systems Engineering
Mechanical Engineering
ISBN:9781118170519
Author:Norman S. Nise
Publisher:WILEY
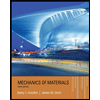
Mechanics of Materials (MindTap Course List)
Mechanical Engineering
ISBN:9781337093347
Author:Barry J. Goodno, James M. Gere
Publisher:Cengage Learning
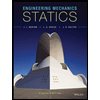
Engineering Mechanics: Statics
Mechanical Engineering
ISBN:9781118807330
Author:James L. Meriam, L. G. Kraige, J. N. Bolton
Publisher:WILEY
Thermodynamic Availability, What is?; Author: MechanicaLEi;https://www.youtube.com/watch?v=-04oxjgS99w;License: Standard Youtube License