PRINT COMPANION ENGINEER THERMO
9th Edition
ISBN: 9781119778011
Author: MORAN
Publisher: WILEY
expand_more
expand_more
format_list_bulleted
Concept explainers
Question
Chapter 4, Problem 4.58P
To determine
The exit temperature of each steam.
Expert Solution & Answer

Want to see the full answer?
Check out a sample textbook solution
Students have asked these similar questions
Question 20
Separate streams of steam and air flow through the turbine and heat exchanger arrangement shown in the
figure below, where mass flow rate m5 is 2000 kg/min and power output Wt1 is 12,000 kW. Steady-state
operating data are provided on the figure. Heat transfer with the surroundings can be neglected, as can all
kinetic and potential energy effects. m5
Steam
in
Turbine
T₁ = 600°C
P₁ = 20 bar
Determine:
(a) T3, in K.
W₁l
T₂ = 400°C
P2= 10 bar
>
6
VT6 = 1200 K
P6 = 1 bar
kW
P3= 10 bar
T3=?
www
tw
Heat exchanger
Turbine
2
Air in
(b) the power output of the second turbine, in kW.
W₁2=?
T5 = 1500 K
-5 P5= 1.35 bar
m5
T₁ = 240°C
P4 = 1 bar
Determine the temperature of the entering steam, in oC.For the overall heat exchanger as the control volume, what is the rate of heat transfer, in kW. Step by step solution please thank you.
Steady-state operating data are provided for a compressor and heat exchanger in the figure below. The power input to the compressor is 50 kW. As shown in the figure, nitrogen (N2) flows through the compressor and heat exchanger with mass flow rate of 0.25 kg/s. The nitrogen is modeled as an ideal gas. A separate cooling stream of helium, modeled as an ideal gas with k=1.67, also flows through the heat exchanger. Stray heat transfer and kinetic and potential energy effects are negligible.
Find:
a) Enthalpy change of Nitrogen from inlet to the compressor and exit from Heat exchanger, ( ℎ1-ℎ3) in kJ/kg,
b) Enthalpy change of Helium from inlet to and exit from Heat exchanger, (ℎ5-ℎ4) in kJ/kg,
c) Mass flow rate of the helium in kg/s.
Chapter 4 Solutions
PRINT COMPANION ENGINEER THERMO
Ch. 4 - Prob. 4.1ECh. 4 - Prob. 4.2ECh. 4 - Prob. 4.3ECh. 4 - Prob. 4.4ECh. 4 - Prob. 4.5ECh. 4 - Prob. 4.6ECh. 4 - Prob. 4.7ECh. 4 - Prob. 4.8ECh. 4 - Prob. 4.9ECh. 4 - Prob. 4.10E
Ch. 4 - Prob. 4.11ECh. 4 - Prob. 4.12ECh. 4 - Prob. 4.13ECh. 4 - Prob. 4.14ECh. 4 - Prob. 4.15ECh. 4 - Prob. 4.1CUCh. 4 - Prob. 4.2CUCh. 4 - Prob. 4.3CUCh. 4 - Prob. 4.4CUCh. 4 - Prob. 4.5CUCh. 4 - Prob. 4.6CUCh. 4 - Prob. 4.7CUCh. 4 - Prob. 4.8CUCh. 4 - Prob. 4.9CUCh. 4 - Prob. 4.10CUCh. 4 - Prob. 4.11CUCh. 4 - Prob. 4.12CUCh. 4 - Prob. 4.13CUCh. 4 - Prob. 4.14CUCh. 4 - Prob. 4.15CUCh. 4 - Prob. 4.16CUCh. 4 - Prob. 4.17CUCh. 4 - Prob. 4.18CUCh. 4 - Prob. 4.19CUCh. 4 - Prob. 4.20CUCh. 4 - Prob. 4.21CUCh. 4 - Prob. 4.22CUCh. 4 - Prob. 4.23CUCh. 4 - Prob. 4.24CUCh. 4 - Prob. 4.25CUCh. 4 - Prob. 4.26CUCh. 4 - Prob. 4.27CUCh. 4 - Prob. 4.28CUCh. 4 - Prob. 4.29CUCh. 4 - Prob. 4.30CUCh. 4 - Prob. 4.31CUCh. 4 - Prob. 4.32CUCh. 4 - Prob. 4.33CUCh. 4 - Prob. 4.34CUCh. 4 - Prob. 4.35CUCh. 4 - Prob. 4.36CUCh. 4 - Prob. 4.37CUCh. 4 - Prob. 4.38CUCh. 4 - Prob. 4.39CUCh. 4 - Prob. 4.40CUCh. 4 - Prob. 4.41CUCh. 4 - Prob. 4.42CUCh. 4 - Prob. 4.43CUCh. 4 - Prob. 4.44CUCh. 4 - Prob. 4.45CUCh. 4 - Prob. 4.46CUCh. 4 - Prob. 4.47CUCh. 4 - Prob. 4.48CUCh. 4 - Prob. 4.49CUCh. 4 - Prob. 4.50CUCh. 4 - Prob. 4.51CUCh. 4 - Prob. 4.1PCh. 4 - Prob. 4.2PCh. 4 - Prob. 4.3PCh. 4 - Prob. 4.4PCh. 4 - Prob. 4.5PCh. 4 - Prob. 4.6PCh. 4 - Prob. 4.7PCh. 4 - Prob. 4.8PCh. 4 - Prob. 4.9PCh. 4 - Prob. 4.10PCh. 4 - Prob. 4.11PCh. 4 - Prob. 4.12PCh. 4 - Prob. 4.13PCh. 4 - Prob. 4.14PCh. 4 - Prob. 4.15PCh. 4 - Prob. 4.16PCh. 4 - Prob. 4.17PCh. 4 - Prob. 4.18PCh. 4 - Prob. 4.19PCh. 4 - Prob. 4.20PCh. 4 - Prob. 4.21PCh. 4 - Prob. 4.22PCh. 4 - Prob. 4.23PCh. 4 - Prob. 4.24PCh. 4 - Prob. 4.25PCh. 4 - Prob. 4.26PCh. 4 - Prob. 4.27PCh. 4 - Prob. 4.28PCh. 4 - Prob. 4.29PCh. 4 - Prob. 4.30PCh. 4 - Prob. 4.31PCh. 4 - Prob. 4.32PCh. 4 - Prob. 4.33PCh. 4 - Prob. 4.34PCh. 4 - Prob. 4.35PCh. 4 - Prob. 4.36PCh. 4 - Prob. 4.37PCh. 4 - Prob. 4.38PCh. 4 - Prob. 4.39PCh. 4 - Prob. 4.40PCh. 4 - Prob. 4.41PCh. 4 - Prob. 4.42PCh. 4 - Prob. 4.43PCh. 4 - Prob. 4.44PCh. 4 - Prob. 4.45PCh. 4 - Prob. 4.46PCh. 4 - Prob. 4.47PCh. 4 - Prob. 4.48PCh. 4 - Prob. 4.49PCh. 4 - Prob. 4.50PCh. 4 - Prob. 4.51PCh. 4 - Prob. 4.52PCh. 4 - Prob. 4.53PCh. 4 - Prob. 4.54PCh. 4 - Prob. 4.55PCh. 4 - Prob. 4.56PCh. 4 - Prob. 4.57PCh. 4 - Prob. 4.58PCh. 4 - Prob. 4.59PCh. 4 - Prob. 4.60PCh. 4 - Prob. 4.61PCh. 4 - Prob. 4.62PCh. 4 - Prob. 4.63PCh. 4 - Prob. 4.64PCh. 4 - Prob. 4.65PCh. 4 - Prob. 4.66PCh. 4 - Prob. 4.67PCh. 4 - Prob. 4.68PCh. 4 - Prob. 4.69PCh. 4 - Prob. 4.70PCh. 4 - Prob. 4.71PCh. 4 - Prob. 4.72PCh. 4 - Prob. 4.73PCh. 4 - Prob. 4.74PCh. 4 - Prob. 4.75PCh. 4 - Prob. 4.76PCh. 4 - Prob. 4.77PCh. 4 - Prob. 4.78PCh. 4 - Prob. 4.79PCh. 4 - Prob. 4.80PCh. 4 - Prob. 4.81PCh. 4 - Prob. 4.82PCh. 4 - Prob. 4.83PCh. 4 - Prob. 4.84PCh. 4 - Prob. 4.85PCh. 4 - Prob. 4.86PCh. 4 - Prob. 4.87PCh. 4 - Prob. 4.88P
Knowledge Booster
Learn more about
Need a deep-dive on the concept behind this application? Look no further. Learn more about this topic, mechanical-engineering and related others by exploring similar questions and additional content below.Similar questions
- Parts A-C have already been answered in a previous question I sub A counterflow heat exchanger operates at steady state while being well-insulated from the surroundings with air and ammonia flowing in separate streams. Ammonia enters at state 1 with -30°C and a quality of 30% and exits at state 2 as saturated vapor at -30°C. Air enters at state 3 with pressure 1 bar and temperature 295 K and exits at state 4 with pressure 1 bar and temperature 265 K. The flow rate of air is 10 kg/s. Ignore kinetic and potential energy effects, and take the dead state as 1 bar and 300 K. a. Describe the heat transfer inside the heat exchanger (what is transferring heat to what?) b. Determine the specific enthalpy of each state, in kJ/kg. c. Determine the mass flow rate of ammonia, in kg/s. d. Determine the rate of exergy destruction within the heat exchanger, in kW.e. Devise and evaluate an exergetic efficiency for the heat exchanger.arrow_forwardExpert Q&A A counterflow heat exchanger operates at steady state while being well-insulated from the surroundings with air and ammonia flowing in separate streams. Ammonia enters at state 1 with -20°C and a quality of 20% and exits at state 2 as saturated vapor at -20°C. Air enters at state 3 with pressure 1 bar and temperature 295 K and exits at state 4 with pressure 1 bar and temperature 265 K. The flow rate of air is 10 kg/s. Ignore kinetic and potential energy effects, and take the dead state as 1 bar and 300 K. a. Sketch states 1 and 2 on a T-s diagram, including the liquid-vapor dome. b. Describe the heat transfer inside the heat exchanger (what is transferring heat to what?) c. Determine the specific enthalpy of each state, in kJ/kg. d. Determine the mass flow rate of ammonia, in kg/s. e. Determine the rate of exergy destruction within the heat exchanger, in kW. f. Devise and evaluate an exergetic efficiency for the heat exchanger. Donearrow_forwardSeparate streams of air and water flow through the compressor and heat exchanger arrangement shown in the figure below, where m, 0.6 kg/s and To = 30°C. Steady-state operating data are provided on the figure. Heat transfer with the surroundings can be neglected, as can all kinetic and potential energy effects. The air is modeled as an ideal gas. 1 Air P₁ = 1 bar T₁ = 300 K m Compressor A P2=3 bar -2 T₂=600 K Determine: WEVA ܒܝ www wwww +6 T6, P6-Ps P₁ = 9 bar T₁=800 K Compressor B 3- P3 P2 T₁=450 K Heat exchanger 5+ 4 Water T5= 20°C Ps= 1 bar (a) the total power for both compressors, in kW. (b) the mass flow rate of the water, in kg/s. WevB =arrow_forward
- Question 32arrow_forwardSeparate streams of steam and air flow through the turbine and heat exchanger arrangement shown in the figure below, where ins = 1500 kg/min and W;1 = 8,000 kW,. Steady-state operating data are provided on the figure. Heat transfer with the surroundings can be neglected, as can all kinetic and potential energy effects. Wn W2 = ? Turbine Turbine 2 P3 = 10 bar T3 =? T = 240°C T2 = 400°C P2= 10 bar P4=1 bar www Steam in 2 T = 600°C PI = 20 bar Ts = 1500 K -5 Ps = 1.35 bar 9. Heat exchanger V T6 = 1200 K P6 = 1 bar Air in Determine: (a) T3, in K. (b) the power output of the second turbine, in kW.arrow_forward7.80 Figure Pz.80 shows an insulated counterflow heat exchanger with carbon dioxide (CO2) and air flowing through the inner and outer channels, respectively. The figure provides data for operation at steady state. The heat exchanger is a component of an overall system operating in an arctic region where the average annual ambient temperature is 20°F. Heat transfer between the heat exchanger and its surroundings can be ignored, as can effects of motion and gravity. Evaluate for the heat exchanger a. the rate of exergy destruction, in Btu/s b. the exergetic efficiency given by Eq. Z27. Let To 20°F, po 1 atm Insulation Mair=1 lb/s T3 500°R 3 P3=50 lbf/in.2 T4=720°R P4=40 lbf/in.2 + Air CO2 mco=2 lb/s T-1200 R P=18 lbf/in.2 T2=1100°R P2= 14.7 lbf/in.2 FIGURE P7.80arrow_forward
- 4.30 Refrigerant 134a enters a heat exchanger operating ai steady state as a superheated vapour at 10 bars. 60°C. where it is cooled and condensed to saturated liquid at 10 bars. The mass flow rate of the refrigerant is 10 kg/min. A separate stream of air enters the heat exchanger at 37°C with a mass flow rate of 80 kg/min. Ignoring heat transfer from the outside of the heat exchanger and neglecting kinetic and potential energy effects, determine the exit air temperature, in °C.arrow_forwardSeparate streams of steam and air flow through the turbine and heat exchanger arrangement shown in the figure below, where air enters location 5 at a rate of 1000 kg/min. The left turbine (Turbine 1) is able to produce 12,000 kW of power. Steady-state operating data are provided on the figure. Heat transfer with the surroundings can be neglected, as can all kinetic and potential energy effects. W2 = ? Turbine Turbine 2 P3 = 10 bar T3 = ? T2 = 400°C_ P2= 10 bar T = 240°C P4 = 1 bar Steam in P1 = 20 bar +6 T = 600°C www T5 = 1500 K -5 Ps = 1.35 bar Heat exchanger V T = 1200 K P6 = 1 bar Air in Determine: T3, in °C. • the mass flow rate of steam at 1, in kg/s. • the power output of the second turbine, in kW. • the magnitude of heat transfer between the steam and air, in kW. • the direction of the heat transfer (i.e., to the steam or from the steam).arrow_forwardEx Clearly please , use table to solvearrow_forward
- Need help please :)arrow_forwarduse table Table Derive expressions for the heat absorbed by the system for each of the following classes of reversible processes for one mole of an idea gas: (a) Case 1: Isothermal change in pressure (b) Case 2: Isobaric change in volume Hint: Case 1 with S = S(T,P); Case 2 with S = S(P,V) (c) Case 3: Isochoric (constant volume) change in temperature dV=Va dT-VB AP dS=CT dT-Va dP dU= (Cp-PVα) dT + V(PB - Ta) dP dH=Cp dT +V(I-Ta) dP dF=-(S+PVa) dT + VPB dP dG=-S dT + V dParrow_forward1. For the pasteurization process, the cold milk with a 4°C temperature value enters the first heat exchanger system. The milk exits the first eat exchanger at 27 °C and enter the 2nd heat exchanger system and exit 54° C. Finally, the milk exit from the 3rd heat exchanger at 72°C (pasteurization temperature). The heat transfer is conducted by steam. The modeling procedures for system controlling is performed based on the initial cold milk temperature. For this system, a. Define process control elements and process dynamic. b. Feed forward or feedback system why? c. How could you express transfer function for this systemarrow_forward
arrow_back_ios
SEE MORE QUESTIONS
arrow_forward_ios
Recommended textbooks for you
- Elements Of ElectromagneticsMechanical EngineeringISBN:9780190698614Author:Sadiku, Matthew N. O.Publisher:Oxford University PressMechanics of Materials (10th Edition)Mechanical EngineeringISBN:9780134319650Author:Russell C. HibbelerPublisher:PEARSONThermodynamics: An Engineering ApproachMechanical EngineeringISBN:9781259822674Author:Yunus A. Cengel Dr., Michael A. BolesPublisher:McGraw-Hill Education
- Control Systems EngineeringMechanical EngineeringISBN:9781118170519Author:Norman S. NisePublisher:WILEYMechanics of Materials (MindTap Course List)Mechanical EngineeringISBN:9781337093347Author:Barry J. Goodno, James M. GerePublisher:Cengage LearningEngineering Mechanics: StaticsMechanical EngineeringISBN:9781118807330Author:James L. Meriam, L. G. Kraige, J. N. BoltonPublisher:WILEY
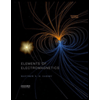
Elements Of Electromagnetics
Mechanical Engineering
ISBN:9780190698614
Author:Sadiku, Matthew N. O.
Publisher:Oxford University Press
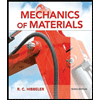
Mechanics of Materials (10th Edition)
Mechanical Engineering
ISBN:9780134319650
Author:Russell C. Hibbeler
Publisher:PEARSON
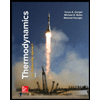
Thermodynamics: An Engineering Approach
Mechanical Engineering
ISBN:9781259822674
Author:Yunus A. Cengel Dr., Michael A. Boles
Publisher:McGraw-Hill Education
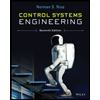
Control Systems Engineering
Mechanical Engineering
ISBN:9781118170519
Author:Norman S. Nise
Publisher:WILEY
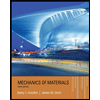
Mechanics of Materials (MindTap Course List)
Mechanical Engineering
ISBN:9781337093347
Author:Barry J. Goodno, James M. Gere
Publisher:Cengage Learning
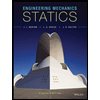
Engineering Mechanics: Statics
Mechanical Engineering
ISBN:9781118807330
Author:James L. Meriam, L. G. Kraige, J. N. Bolton
Publisher:WILEY
How Shell and Tube Heat Exchangers Work (Engineering); Author: saVRee;https://www.youtube.com/watch?v=OyQ3SaU4KKU;License: Standard Youtube License