BIOL368 Lab manual COVID-19 edition Week 1
pdf
keyboard_arrow_up
School
Concordia University *
*We aren’t endorsed by this school
Course
368
Subject
Biology
Date
Jan 9, 2024
Type
Pages
38
Uploaded by biogeekconcordia
BIOL 368 Lab Manual Week 1 1 Week 1
Project 1: Dilution, Pipetting & Spectrophotometer Introduction
Many scientific experiments are designed to give quantitative data; that is numbers obtained by various techniques. It is important to recognize the limitations of a measurement technique. You should ask the following questions about each technique you use. What is the sensitivity of the technique; that is, what is the smallest accurate number that can be determined? What is the upper limit of the technique? Are there any factors that interfere with obtaining accurate measurements? How reproducible are the measurements? A. Dilutions:
Often suspensions and solutions available in a laboratory are more concentrated than needed for an experiment. Dilutions can be confusing because we refer to them in different ways. This table summarizes some of the common ways of describing dilutions. Note that the volumes used depend on the requirements of the experiment and limitations of measuring instruments but that the units must be the same. For example, a 1/5 dilution could be 1 µL of the sample with 4 µL of dilution fluid or it could be 10 L of the sample with 40 L of dilution fluid. Table 1.1. Dilution examples Dilution Volume of Sample Volume of Diluting Fluid* Total Volume 1/5 1 4 5 3 in 17 3 14 17 6-fold 1 5 6 10
-6
(1/10
6
) 1 999,999 1,000,000** *-The diluting fluid to be used depends on the sample. An aqueous solution is diluted with water, whereas a suspension of cells (bacteria, blood, etc.) is normally diluted with an isotonic saline solution. **-This requires serial dilution
(see below). Serial dilutions
: Very large dilutions (for example, 10
-6
) are normally obtained by making a series of smaller dilutions (serial dilutions) rather than one large dilution. Generally, serial dilutions use 10- or 100-fold dilutions to obtain the desired final concentration. For example, a thousand-fold (10
-3
) dilution can be made by diluting the sample 10-fold (10
-1
), then diluting the 10
-1
dilution 10-fold (the sample has now been diluted 100-fold) and finally diluting the10
-1
another 10-fold. (1000 fold dilution = 10
-1
x 10
-1
x 10
-1
= 10
-3
). This is an example of 10-fold serial dilution, where 10-fold dilutions are repeated to obtain a higher degree of dilution. Do not get confused with 10-
fold dilution, where 10-fold dilution is made only once. Some procedures require serial dilution using a dilution factor other than 10. For example, in Project 7, you will need to create 5-fold serial dilutions (1/5, 1/25, 1/125, etc.).
BIOL 368 Lab Manual Week 1 2 You should be able to calculate how to dilute a solution of known concentration to give a solution with a desired lower concentration. You should also be able to calculate the concentration of a stock solution given measurements made on diluted samples of that solution. Reminder: Online quiz 2 (Moodle) is based on dilution problems. Don’t forget to complete it before the deadline. B. Pipettors:
You will use micropipettors and tips for most of your measurements of volume in this laboratory. You will use four sizes of micropipettors, which will handle volumes ranging from 0.5 µL to 1000 µL (1 mL). Familiarize yourself with the measurement ranges of the pipettors (written on the top of the instrument), the setting of desired volumes and the appropriate-sized tips. Table 1.2. Pipettor ranges. Range of Pipettor (µL)
P10 0.5 - 10 P20 2 - 20 P200 20 - 200 P1000 100 - 1000 Different brands of pipettors may have slightly different ranges. Check specifications whenever you use a new pipettor. Watch the demonstration video about micropipette before this part Procedures: A.
Pipetting exercise First, practice pipetting without any liquid. Then practice with water. 1.
Place an appropriate pipette tip (a blue tip for P1000, a yellow or clear tip for P200 and P20) to the pipette. 2.
Dip the tip into liquid. Do not immerse the entire tip. Keep the pipette near vertical with the tip down when there is a sample in the tip. 3.
When you measure, depress the piston until you feel resistance. Do not depress all the way. 4.
Release the piston. If you release too fast, the liquid will splash in the tip and can cross-
contaminate liquid. It can also damage the pipette ($$). 5.
Take the tip out of the liquid. Remove droplets on the outside of the pipet tip by gently touching the container. Check if there is any leakage. Check if the liquid in the tip looks like the right volume. 6.
Dispense the content by pressing the piston all the way (as far as it can go). 7.
Once you are comfortable with pipettes, determine the accuracy of your pipettes and your pipetting skill by pipetting distilled water.
BIOL 368 Lab Manual Week 1 3 8.
You need to use a digital scale for this exercise. Place an empty plastic weighing boat on the scale and close the door. Press O/T. You should see zeros on display. 9.
Open the door. Pipette the following amount of water (Table 1.3) into the weighing boat. Close the door and wait until the value stabilizes. Write down the weight in mg. Enter the value in Table 1.3. Calculate the error rate right away and make an entry. Table 1.3. The weight of water observed vs. expected Pipette Volume (µL)
Expected weight (mg)* Observed weight (mg) Error (%) P200 56.5 P1000 812 * Calculate expected weight, assuming that the density of water is 1 kg/L. Since the density of water is constant at a constant temperature, the error is mostly due to instrumental and human errors. If the error rate is more than 2 %, try it again. If the error rate is more than 2 % for the second time, ask your TA to troubleshoot your pipetting skill. If both you and your TA give large error rates, the pipette may need to be calibrated. B.
Spectrophotometer exercise Introduction: Spectrophotometers measure the amount of light of a specific wavelength that is transmitted or absorbed by a sample. They are used in a wide variety of applications. A common use is for measuring the concentration of solute in various types of solutions. The absorbance of the solution is directly proportional to the amount of solute present. However, the proportionality between solute concentration and absorbance is lost at high absorbance range. For our spectrophotometer, values higher than 3 and lower than 0.1 are not reliable. You are given a Coomassie Blue stock solution, which contains a known amount of the Coomassie blue dye dissolved in 50 % ethanol. Use this solution to create a standard curve showing the relationship between Coomassie Blue concentrations and OD
540
. OD
540
is an abbreviation of the optical density at 540 nm. In our spectrophotometer, optical density is the same as absorbance. Thus it is interchangeable with A
540
, the absorbance at 540nm. You are also given a solution labelled as ‘Unknown.’ This solution contains an unknown concentration of Coomassie Blue dye. You need to determine the concentration using the standard curve you created.
Your preview ends here
Eager to read complete document? Join bartleby learn and gain access to the full version
- Access to all documents
- Unlimited textbook solutions
- 24/7 expert homework help
BIOL 368 Lab Manual Week 1 4 1.
The concentration of the stock solution of Coomassie blue you are given is 200 µg/mL. You will prepare dilutions of this solution to give five samples covering the concentration range of 20-100 µg/mL (Final volume 1 mL.): 20, 40, 60, 80 and 100 µg/mL. Prelab Assignment: Calculate how much diluent (50 % ethanol) and Coomassie blue solution you need to add.
Use Table 1.4. for calculation. This table should be entered in your lab book.
Table 1.4. Coomassie Blue dilutions and concentrations. Final Concentration Of Coomassie Blue (µg/mL) Volume (µL) Of 50 % ethanol Volume (µL) of Coomassie Blue Stock Solution Total volume (µL) 20 40 60 80 100 2.
You will need six microfuge tubes. Use a pipette to pipette the diluent (50 % ethanol) and the dye solution into the tubes, as indicated in the table. 3.
In addition, create a four-fold dilution of the Unknown solution. Use this dilution for measuring OD. Perform these dilutions at your workbench before transferring the contents to spectrophotometer cuvettes. IMPORTANT! Mix the stock solution before pipetting. Mix the dilutions after pipetting.
4.
Take your samples and the diluent to a spectrophotometer. There are cuvettes beside each spectrophotometer. Check that the cuvettes are clean and scratch-free on the measuring surfaces (smooth sides). Transfer your samples into the cuvettes. Fill the first cuvette with the diluent. This is your blank. 5.
Please do not start unless you see the demonstration by a TA. Set the wavelength to 540 nm. Place all the cuvettes in the same orientation using the etched arrow on the top of the cuvettes. Place the blank cuvette in the blue cuvette holder. Place the cuvettes containing your sample in black holders. Zero the instrument on the blank cuvette. And then, read the absorbance of your samples and record them in your lab book. 6.
Once finished, empty the cuvettes into a specially provided waste container. Carefully rinse both the interior and exterior of the cuvettes with distilled water. Leave them upside down to dry. 7.
Before leaving the spectrophotometer, check your pipettes to see if you have fluid in or on the barrels. If the end is blue or wet, you are not pipetting correctly. You MUST ask your TA for assistance with both technique and how to clean your pipettors. The proper pipetting technique
BIOL 368 Lab Manual Week 1 5 is one of the crucial aspects of good lab work. A bad pipetting technique will yield inconsistent data, which are impossible to interpret. 8.
Copy your data to Week 1 Datasheet. Submit the datasheet to your TA. IMPORTANT:
Datasheet will not be returned to you. You must enter all your data in your lab book. 9.
Tip: Before leaving the lab, check with the TA to see if your OD values are similar to the rest of the class. If they are clear differences you may be asked to repeat the experiment. The experiment can be repeated relatively quickly. But if your values look like outliers, it will take a lot longer to explain the discrepancies in your lab report.
The following activity is to practice aseptic techniques. Aseptic techniques are used whenever we handle bacterial cultures to avoid contamination
. C.
Pouring agar into plates under the aseptic condition Watch the demonstration video about fire safety before this part 1. (Done anytime during the lab) Clear the area to remove anything flammable near the burner. (1) Practice turning on and off the alcohol burner. Repeat again so that your body remembers the procedure. (2) Practice turning on and off the Bunsen burner. If you do not have a Bunsen burner on your bench, ask your TA which one you can use for practice. Bunsen burner is tricker than the alcohol burner. Repeat until you are comfortable with the burner. 2. (Done at the end of the lab) Before completing this part, you should have completed all other work and cleaned up the bench area. Used tips and tubes should be in a proper disposal area. Pipettes and other equipment should be placed back in the racks, shelves or the storage box. Watch the demonstration video about pouring media into plates before this part 3. Bring a flask containing melted agar to your bench. The agar is kept in a warm water bath to prevent solidifying. Agar should be cooled to about 50 o
C before pouring. At this temperature, you should be able to handle the bottle with your bare hands. (If agar cools down too much and solidify, microwave it to melt.) 3. Wash hands thoroughly with soap and dry with a paper towel. Wipe the bench area with a disinfectant such as ethanol. Let the benchtop dry. 4. Turn on the Bunsen burner or the alcohol burner. If you have both on your bench, use the Bunsen burner. If you have only the alcohol burner, use the alcohol burner. Feel the area of warm air. This is your aseptic working area. You should not work too close to the fire, but you should not work too far away. 5. Take out plastic Petri dishes from the bag and place them on your bench.
BIOL 368 Lab Manual Week 1 6 6. Take off the lid of the flask and hover the mouth of the flask over the flame. (This creates outward air movement and microbes are less likely to enter into the flask) 6. Pour agar medium into plastic sterile Petri dishes. Lift the lid halfway and pour media just enough to cover the bottom of the plate (about 25 mL). Avoid introducing bubbles. Close the lid. Let the agar to cool and solidify. Do not move plates! 7. Since it will take ~ 30 min or so for agar to solidify, you can leave the lab at this point. Done by TA: When plates are cool, put them upside down and add media labels. Place plates on a designated tray for storage. Please follow the direction in BIOL368 Lab Report Guideline and Project 1 grading scheme. End of experiments.
Have you cleaned up your bench? Have you given your datasheet to your TA? Analysis of data: spectrophotometer exercise (done at home):
1.
Plot your data on a graph of the absorbance measured (y-axis) versus the concentration of Coomassie blue (x-axis). Graphs must be made using spreadsheet software such as Excel. If you are not sure how to use Excel, check Excel self-tutorials at Microsoft Office training https://support.microsoft.com/en-ca/office or Microsoft Office 365 training https://support.microsoft.com/en-ca/microsoft-365. 2.
When you create a graph, select the ‘scatter plot’ not ‘line graph.’ 3.
Create a linear regression line using the ‘trendline’ function. Select the data and right-click the mouse. Select ‘Add Trendline.’ Select ‘Linear,’ ‘Display Equation on the chart,’ ‘Display R-squared value on the chart.’ The r-squared value will tell you how closely the data fit the regression line. 4.
Add axis titles. Add units if necessary. Copy and paste the graphs into your report file. 5.
Determine the Coomassie blue concentration of the Unknown solution using your data. Don’t forget to calculate the concentration of the original solution. 6.
Download section data from Moodle (as your TA if you can’t find it). Calculate the section average and corresponding standard deviation for each concentration. You can do this easily in Excel using the ‘Function’ tool. Plot another graph using the section average. Add error bars using the standard deviation you calculated. 7.
Describe the two graphs. Is the absorbance proportional to the concentration? How well do standard curves fit the linear regression line? How do your data compare with that from the rest of your section? Does your section data contain an outlier? Discuss data variation in the section data. Compare against pipetting exercise (Table 1.3). Can you attribute the OD value variation solely to pipetting errors and variations? Read BIOL368 Lab Report Guideline and Project 1 grading scheme before writing the lab report
Your preview ends here
Eager to read complete document? Join bartleby learn and gain access to the full version
- Access to all documents
- Unlimited textbook solutions
- 24/7 expert homework help
BIOL 368 Lab Manual Week 2 7 Week 2 (at home) Project 2: Sterilization and aseptic technique Introduction
: Generally, we work with pure cultures of microorganisms; that is, all of the cells in a population are descendants of a single cell. To maintain pure cultures, it is essential that we avoid introducing foreign microorganisms into our pure cultures. Since microorganisms are prevalent in our environment, we must use special precautions to prevent them from contaminating our pure cultures. A.
Sterilization All media, solutions, and glassware must be sterilized. Sterilization involves the complete elimination of all living organisms on, or in, a given material. A number of sterilization methods are routinely used depending on materials. 1.
Autoclaving Glassware, heat resistant plasticware and many solutions can be sterilized in an autoclave machine. The autoclave is essentially a large pressure cooker in which steam is generated under pressure, so the temperature of the steam is 121°C. This temperature under wet conditions will kill even the most resistant microorganisms. Materials are kept at 121 o
C for at least 15 min. Most of the materials in the lab are sterilized by autoclaving. 2.
Radiation Heat sensitive plastics are sterilized using radiation (gamma-ray, X-ray or UV) or ethylene oxide. Manufacturers normally sterilize these materials because both methods are hazardous and can only be used under very controlled conditions. 3.
Filter sterilization Solutions containing compounds that are heat-labile (i.e. broken down at high temperatures) are sterilized by filtration through sterile filters that retain bacteria and other microorganisms. We commonly use nitrocellulose filters that have pores with a diameter of 0.22 µm. E. coli
is a rod-
shaped organism 5 µm by 1 µm and is thus easily retained on the filter, as are most other microorganisms. However, some viruses can pass through these filters. B.
Aseptic techniques Aseptic techniques are used whenever we handle bacterial cultures to avoid contamination. 1.
Wipe the lab bench with a disinfectant This is to minimize the number of microorganisms in the work area and reduce the risk of contamination. Use disinfectants such as 70 % ethanol or dilute solutions of disinfecting soap. Disinfectants kill most microorganisms but do not sterilize the area. 2.
Bunsen burner When you open anything that is sterile (tubes, tips, plates, etc.) turn on a Bunsen burner on the bench. The flame generates upward air movement and minimizes the chance of microorganisms landing on to your sterile media. Do not put flammable materials (notebooks, etc.) near the flame. Keep your long hair tied back!
BIOL 368 Lab Manual Week 2 8 3.
Opening lids Minimize the time of opening lids. The mouths of glass
tubes or flasks are heated briefly in the flame. The heat causes convection currents that move away from the mouth of the tube and make it difficult for microorganisms in the air to fall into the tube. The mouths of plastic tubes need not be heated as you will risk melting them. Sterile objects such as test tube caps and pipette tips should not touch non-sterile objects such as the lab bench, the outside of tubes, your fingers, etc. If that happens, exchange the contaminated object with a sterile one. C.
Biohazard discards Any materials containing microbial cultures must be sterilized before discarding. Therefore, we have special procedures for biohazard garbage. •
Pipette tips & microfuge tubes: Discard into yellow plastic biohazard containers on the lab bench. •
Agar plates containing microbial cultures: Discard into orange biohazard bags. •
Glass culture tubes containing liquid microbial cultures: Keep in a tube rack. NEVER discard the culture into the sink. The technician autoclaves these materials after the class. A. Week 2 at-home assignment: The ubiquity of microorganisms
Complete this portion and upload it to Moodle before midnight of the Week 2 lab day. When working with any microorganisms, it is important to be aware that contamination can occur from many different sources. In this exercise, you will expose agar plates to contamination in a number of ways to illustrate this. Obtain samples from different places and inoculate them on either Tryptic Soy Agar (TSA) or malt extract agar. TSA is optimized for the growth of bacteria because bacteria require high nitrogen (N) content and low carbon (C) for growth. Bacteria grow best under neutral to slightly basic conditions. Malt extract agar is optimized for the growth of fungi. Fungi require a high C: N ratio and favour acidic conditions. 1. Design an experiment that identifies microorganisms in the environment. Each student will be given four agar plates: two TSA and two Malt extract agar plates. Make a plan for your experiments using the following table. Each plate will be divided into four sections by drawing on the bottom of the plate. Eight different environmental samples will be inoculated on two plates. The same eight samples should be inoculated on both TSA agar and Malt agar. 2. The following list of suggestions can help you decide. Packaged sterile swabs are available if needed, as well as sterile water for moistening the swabs for use on dry surfaces. a)
Place your dirty fingers (of each hand) on the agar. b)
Wash hands with soap, then place your clean fingers on the agar. c)
Place a hair on your head on the agar surface.
BIOL 368 Lab Manual Week 2 9 d)
Swab inside of your mouth. e)
Swab around the sink. f)
Swab dust from a dusty shelf onto the agar surface.
g)
Swab your belongings such as cell phones, shoes, etc.
h)
Drop a small aliquot of bacterial culture on the bench. Swab the spot. Then spray 70 % ethanol and wait for 5 min. Swab the same spot. (If you wish, you can bring a commercial disinfectant and test the efficacy.)
TIP: If your sample is from the room environment, incubate plates at room temperature. If your sample is from inside of the body, such as mouth swab, incubate at 37 o
C. If your sample is from the outer surface of the body, such as skin & hair, incubate at 30 o
C. All samples on the same plate should be incubated at the same temperature. Table 2.1. The ubiquity fo microorganism experimental design Please create a table similar to this in your document. Sample Description Incubation temperature Plate 1 1 2 3 4 Plate 2 5 6 7 8 3. Describe what you expect from these experiments. What type of microbes are commonly found in the environment you are testing? You'll have to check in the literature. Follow proper citation guidelines.
Your preview ends here
Eager to read complete document? Join bartleby learn and gain access to the full version
- Access to all documents
- Unlimited textbook solutions
- 24/7 expert homework help
BIOL 368 Lab Manual Week 3 10 Week 3 (Lab) Project 2: Sterilization and aseptic technique (continued) Watch the following demonstration video before coming to the lab. •
Aseptic techniques •
Dilution and serial dilution •
Plating •
Streaking •
Analyze bacterial growth Procedures: Escherichia coli wild-type strain CAG 12033 is used unless otherwise noted.
Use aseptic techniques.
A.
Isolation of pure cultures of bacteria by streaking
The easiest way to obtain pure cultures is to obtain colonies from single cells. The cells in each colony are descendants of the original cell and, thus, are considered a pure culture. To create well-separated single colonies, one must dilute the original culture so that bacterial cells are well separated. Streaking
is a technique used to isolate single colonies (pure cultures) quickly. It is an essential technique in any microbiology/molecular biology labs. Watch the demonstration by the technician or the TAs. They can show you a few different methods. 1.
Obtain a pure E. coli
and an LB agar plate. Plates are placed bottoms up (the bottom is the part with the agar in it) to keep any condensation off the agar. LB is a nutrient rich medium that contains all nutrients required for bacterial growth. 2.
Use a wax crayon or marker to label on the bottom
(not on the lid!) of your plates with your name, date and laboratory section. Write this pertinent information around the edge and not across the bottom. You can also mark the plate to show the starting and stopping points of your streaks. 3.
The pattern used for streaking for single colonies is a matter of personal preference, as long as you understand the purpose of the technique. An example of streaking pattern is shown figure on the right.
BIOL 368 Lab Manual Week 3 11 <Method using a metal loop> 1.
Flame your loop until it is red hot (holding it upwards and away from your face) and let it cool down for a few seconds. Sterilize the whole wire, not just the tip. 2.
Lift the bottom of the E. coli
plate and touch the loop on the agar where there is no bacterial growth. This ensures that the loop is cool enough that it doesn't kill the bacteria. Then touch a single colony of the bacteria lightly. If you can see a blob of bacteria on your loop, you have picked up too many and may not get isolated single colonies. 3.
Close the E. coli
plate and pick up the bottom of a new plate. Spread the loop full of bacteria on the surface of the agar in your plate. Use a light touch, or the loop will cut the agar (Step 1).
4.
Flame your loop again to kill the bacteria on the loop. Cool it in the agar at the side of your plate. Touch the end of the first streak, and spread onto the fresh agar (Step 2). This will dilute the cells on the agar. Sterilize the loop after streaking. 5.
Repeat streaking (Step 3 and 4). Sterilize the loop between streaks. You can stop at the third streaks if you are confident that you can get single colonies by then. Sterilize the loop before putting it away. <Method using a sterile inoculating stick> 1.
Inoculating sticks should not be flamed. They have been sterilized by autoclaving. Take a fresh stick and use it for picking up a colony from the E. coli
plate. Streak the cells onto a new plate (Step 1). 2.
Discard the stick into a biohazard disposal container. Take another sterile stick and use it for second streaking. Touch the end of the first streak and spread onto the fresh agar (Step 2). Discard the stick. Repeat streaking using a fresh stick (Step 3 and 4). 3.
Place your plate upside down on the tray provided for your group. 4.
Plates will be incubated overnight at 37°C and the technician will store them at 4°C until observation can be made. 5.
Your TA will evaluate your streaking plates according to the following criteria. •
Plates are not contaminated •
Two or more single colonies have been isolated If you are not successful, you can try again in Week 5.
BIOL 368 Lab Manual Week 3 12 B.
Viable count. The viable count is a method used to determine the number of bacterial cells in a sample. You will use this Method several more times during this course. Serial dilutions
are the key part of the viable count method. Serial dilutions are used to dilute concentrated suspensions or solutions. In the viable count, a suspension of bacteria is diluted and then aliquots of the dilutions are plated on an agar medium where the cells can grow. If the suspension has been adequately diluted, the cells will be well dispersed on the surface of the agar. Each cell divides and after many generations, a colony containing many cells is visible to the naked eye. The number of colonies is equivalent to the number of cells originally plated. If the sample is not diluted sufficiently a lawn of bacteria covering the entire plate will be observed. In this experiment, we estimate the number of bacteria cells present in a liquid culture of E. coli.
This protocol will tell us the number of viable cells (that is, cells able to grow). Slight modifications of the technique allow us to assay some types of mutant cells in a pure culture of bacteria, or the number of bacterial cells in assorted materials such as hamburger, milk, feces, or pond water. Use of pipettors:
Use pipette tips that have been autoclaved. Open the tip box only in the aseptic environment (i.e. flame on). Pick up the tip by inserting the barrel into the top of the tip. Avoid touching nearby tips to prevent contamination. Do not touch the tip with your hands or gloves. NEVER ENTER THE BARREL OF THE PIPETTOR INTO THE TEST TUBE, ONLY THE TIP OF THE INSTRUMENT IS STERILE. To reach the sample at the bottom of a tube, tilt it until the liquid comes up the side, and you can reach it with the tip of the pipettor. Depress the piston on the pipettor to the first stop. Insert the tip into the sample, and draw the aliquot into the tip by slowly releasing the piston. DO NOT RELEASE THE PISTON TOO FAST.
This will cause the sample to splash up into the barrel of the pipettor resulting in inaccurate measurements, in addition to contaminating and deteriorating the instrument. Dispense the sample into an appropriate tube by slowly depressing the piston all the way.
Sterilization of spreader:
Instruments such as spreaders and forceps are sterilized by flaming with alcohol rather than heating them red hot as you do with your inoculating loop. The burning alcohol effectively incinerates any bacterial or other microorganisms on the instrument but does not have a very hot flame. The instrument is therefore left cooler than if it were heated to red-
hot and cooled down faster as a result. Practice this at least once before proceeding to the experiment. Read the following directions and plan how you are going to carry out each step.
Use aseptic techniques.
Your preview ends here
Eager to read complete document? Join bartleby learn and gain access to the full version
- Access to all documents
- Unlimited textbook solutions
- 24/7 expert homework help
BIOL 368 Lab Manual Week 3 13 <Serial dilution> 1.
You have been provided with a test tube rack, an overnight liquid culture of E. coli
, a sterile MinA salt solution, sterile microfuge tubes, and three LB plates. MinA salt solution is isotonic to bacterial cells. A sterile isotonic saline salt solution may also be used to dilute bacterial cells. Never use water to dilute living cells. It will kill them instantly. 2.
Label seven sterile microfuge tubes 1-7. Label your plates (on the bottom) with your names, lab section, the date, and dilutions (10
-5
, 10
-6
or 10
-7
). 3.
Pipette 900 µL (0.9 mL) of sterile MinA salt solution into each of your sterile microfuge tubes. You can use one sterile pipette tip for all the samples as long as you do not contaminate the tip. If you contaminate the tip by touching non-sterile objects, discard it and use another sterile tip. Discard used tips in a biohazard container. 4.
Use a sterile pipette tip to pipette 100 µL (0.1 mL) of the E. coli culture into tube #1. Mix thoroughly by inverting the tube a few times (or vortexing the tube briefly). Discard the used tip into a tip/microfuge waste container. (Pipette tips and microfuge tubes should be discarded into a biohazard waste can at the end of the lab.) The bacterial culture has now been diluted 10-fold (10
-1
). 5.
Use another sterile pipette tip to transfer 100 µL of cell suspension from tube #1 (10
-1
) to tube #2. Discard the tip. Mix. The cells have now been diluted 100-fold (10
-2
). 6.
Transfer 100 µL from tube #2 to tube #3 (10
-3
). 7.
Repeat for tube #3 to #4 (10
-4
), #4 to #5 (10
-5
), #5 to #6 (10
-6
), and #6 to #7 (10
-7
). Use a new sterile pipette tip for each transfer. <Plating> 8.
Place the LB plate labeled 10
-7
the lid side up. Take 100 µL of cell suspension from tube #7. Pipette the suspension on the surface of the LB plate labeled 10
-7
. Open the lid of petri dish only to pipette the cell suspension and close it immediately. Discard the pipette tip. 9.
Sterilize the spreader: a) Remove the cover from the ethanol dish. b) Dip spreader in ethanol, shake it lightly over the dish to remove excess ethanol. c) PUT BACK THE DISH COVER BEFORE FLAMING SPREADER. This will minimize the risk of ethanol catching fire. If the ethanol dish ignites, just cover it with the lid. (Don't panic.)
BIOL 368 Lab Manual Week 3 14 d) Put spreader in Bunsen flame to ignite ethanol. Remove it from the Bunsen flame as soon as ethanol ignites. Hold the spreader horizontally until the flame dies and allow the spreader to cool for a few seconds. Do not wave it around or blow on it… 10.
Spread the 100 µL of cell suspension on the plate so that it covers the agar uniformly. Keep the plate on the bench while spreading. Turn the plate slowly while you are spreading. After spreading, close the lid and keep the lid side up until all liquid has been absorbed into the agar. 11.
Sterilize the spreader by flaming in ethanol (see above). 12.
Repeat steps 8-11 to plate 100 µL each from tube 6 on the plate labeled 10
-6
, and from tube 5 on plate 10
-5
. Check the pipettors to see if you have fluid in the barrels. The barrels should be dry if you are pipetting correctly
13.
After all the liquid has been absorbed into the agar, tape plates together and place them on the tray. Invert the plates so that the bottoms face up. 14.
The plates will be incubated overnight at 37°C. The technician will store the plates at 4 o
C until observations can be made. 15.
Your TA will evaluate your viable count plates according to the following criteria. •
Plates are not contaminated •
Cells are spread uniformly on the agar •
Cells appear to be properly diluted •
The number of colonies is in the same range as the control plates. If you are not successful, you may repeat the procedure in Week 5. The following procedures describe analyses of bacterial cultures. C. Bacterial cell count by optical density (OD
600
) OD
600
measures the turbidity in bacterial cultures due to light scattering by cell particles and, therefore, approximately correlates with bacterial cell density. 1.
Aseptically make a 10-fold dilution of E. coli
in a microfuge tube. Use LB broth as a diluting liquid (not MinA). The final volume should be 1 mL. 2.
Measure OD
600
of the diluted culture in a spectrophotometer. You don't need to use aseptic techniques at this point. However, pay extra attention not to splash bacteria cells in the spectrophotometer. 3.
Use LB broth as blank. Record the value. Discard cuvettes into the biohazard container. Calculate the cell density of the diluted culture and calculate the cell density of the original culture
,
BIOL 368 Lab Manual Week 3 15 assuming that an E. coli
culture with OD
600
=1 contains 5 x 10
8
cells/mL. Record the value obtained by your partner. Include both values in the lab report. D. Viable cell count (observation & analysis) 1.
Due to the time constraint, you will analyze the vialbe cell count plates prepared by the technician instead of the plates you created. 2.
Examine the plates. Do any of them have isolated colonies? Do any have a bacterial lawn? On plates with isolated colonies are all the colonies the same? 3.
Count the colonies on each plate that has colonies. You can use a tally counter to keep track of the number and mark each colony (
on the bottom of the plate
) with a marking pen. If a plate has very large numbers of colonies you can count a segment of the plate. Compare the numbers on plates -4, -5 and -6. There should be 10 times as many colonies on the plate -5 as on -6 and a similar relationship between the -4 and -5 plates. 4.
Enter your count (raw data) into your lab book and into the datasheet. 5.
Calculate the viable count as follows. Do the calculations of your data while you are in the lab. Check with TA and other groups for the accuracy of your calculation. Viable count calculation The purpose of the viable count is to estimate the number of cells in the culture you assayed. Since single colonies are formed from single cells, the number of colonies (colony forming unit, cfu) should correspond to the number of viable cells in the original culture. Choose the plate having approximately 30-300 colonies to calculate the number of cells in the culture. Plates with low numbers of colonies give a large sampling error, while plate with large numbers of colonies tend to give a low estimate because overcrowding may result in some cells not producing a colony or some colonies resulting from more than one cell. Sample calculation. •
Suppose the -6 plate has 120 colonies. •
This indicates that the 100 µL of10
-6
dilution contained 120 cells. •
This indicates that the 100 µL of undiluted culture should have contained 10
6
times more cells: 120/10
-6
= 120 x 10
6
= 1.20 x 10
8
cfu/100 µL (Keep three significant digits unless noted otherwise.) •
The unit of viable count is cfu/mL. Therefore, you need to convert the value into per mL: 1.20 x 10
8
cfu/ 0.1 mL =1.20 x 10
9
cfu/mL
Your preview ends here
Eager to read complete document? Join bartleby learn and gain access to the full version
- Access to all documents
- Unlimited textbook solutions
- 24/7 expert homework help
BIOL 368 Lab Manual Week 3 16 To summarize:
Viable count (cfu/mL) = # of colonies / dilution /volume in mL Our example: 120 cfu / 10
-6
/ 0.1mL =1.20 x 10
9 cfu/mL Enter the colony count (cfu) and the viable count (cfu/mL) into the datasheet. If you are aware of any problems with your results note them. Compare your results against others. At home: 1.
Download section data from Moodle. Calculate viable count from using Excel. You can enter an appropriate equation into the cell. Copy & paste the equation or use the 'fill' function to repeat analysis for all data. When calculating viable count, do not include the data that had too many or too few colonies on plates. Calculate the average for each lab section and for all the data. 2.
Are the section averages very different from the class average? What is the range of the data (minimum and maximum) for each lab section and for the entire class? Are section rages very different from the class range? How much do individual values deviate from the average? 3.
Compare your viable count data (cfu/mL) with the cell density data (cells/mL) you obtained in the previous week. Are the values consistent? How does the OD
600
data variation compare with the viable count data variation? Are they similar? If there are discrepancies, provide possible reasons. Review of exponential numbers For those of you who have forgotten all you ever knew about working with exponential numbers: 5.67 X 10
6
5,670,000; 5.67 X 10
-6
= 0.00000567 To multiply: Add exponents. a X 10
x
* b X 10
y
= a * b X 10
(x + y)
5 X 10
6
* 4 X 10
4
= 20 X 10
10
= 2.0 X 10
11 To Divide: Subtract exponents. a X 10
x
/ b X 10
y
= a/b X 10
(x-y)
5 X 10
4
/ 2.5 X 10
8
= 2 X 10
-4
Addition and Subtraction: express them all to the same exponent. 2.3 X 10
7
- 3 X 10
6
+ 5.4 X 10
8
=23 X 10
6
- 3 X 10
6
+ 540 X 10
6
=560 X 10
6
= 5.6 X 10
8
BIOL 368 Lab Manual Week 3 17 E. The ubiquity of microorganisms (observation) 1.
Due to the time constrain, you’ll not be able to perform your own experiments. But you need to observe the plates prepared by the technician. 2.
Record the number of fungal and bacterial colonies from each source of contamination. •
Fungal growth can be easily distinguished from bacteria by looking at their surface textures. Fungi are usually "fuzzy" or "hairy" due to mycelial growth on the agar medium. Describe the colony morphology of the bacteria growing on your plate. Use the colony morphology table on the next page as your guide. •
Bacterial genera may be differentiated in two ways: by the cellular morphology which is observed microscopically and by the morphology of isolated colonies grown on an agar plate. Describe the colony morphology of the bacteria growing on your plate by using the information and descriptive vocabulary provided below. 3.
How are colonies grown on TSA and Malt agar plates different? 4.
Example of colony morphology: •
Surface: smooth, rough, mucoid, dry •
Size: pinpoint, small, or large •
Pigment: cream-colored organisms are usually considered to be non-pigmented. Pigments may be purple, red, pink, yellow, brown, blue, grey, etc. Water-soluble pigments diffuse into the media. •
Opacity: transparent, translucent or opaque
BIOL 368 Lab Manual Week 3 18 Demonstration: Growth of E. coli
E. coli
will grow in a large variety of media. These media can be chemically defined (for example, minimal medium) or not (for example, nutrient agar). In addition, the medium can be solidified with agar or can be liquid. To grow E. coli
, the sterile medium is inoculated with E. coli
cells and then is incubated to allow the growth of the culture. The cells start dividing after a period of adaptation to the new conditions (this lag period varies depending on how different the conditions are from those of the inoculum). The cells continue to divide until toxic wastes or lack of nutrients limit their growth when they enter the stationary phase. There are cultures of E. coli
in flasks containing a liquid medium. Compare the appearance of media containing bacteria to that in a flask that was not inoculated. The availability of air is reasonably good in large flasks containing small amounts of the medium. One can also increase aeration by shaking. E. coli
growing at 37°C in liquid culture reaches stationary phase 6-8 hours after inoculation, and the culture will then contain approximately 5 X 10
9
cells/mL. There are also Petri dishes containing solid medium with cultures of E. coli
growing on the surface. The solid medium is the same as the liquid medium except that it has been solidified with agar. Agar is a complex polysaccharide extracted from red algae and is the most commonly used solidifying agent. It produces a stiff transparent gel and is indigestible for most bacteria. Agar solutions melt at 100°C and remain liquid between 100°C and 45°C. Agar solutions solidify at lower temperatures. We purchase empty petri dishes that are sterilized by the manufacturer using gas or radiation. The sterile agar containing medium is then poured into them aseptically. The plates were streaked with E. coli
cells from a pure culture. Observe the heavy bacterial growth near the top of the plate and the isolated colonies near the bottom. A single colony can contain in the order of 10
8
cells. Since the lid of the Petri dish does not fit tightly there is a good gas exchange and growth conditions on the plate are aerobic although cells in the middle of a colony will become limited for oxygen. End of experiments. Have you cleaned up your bench? Have you given your data sheet to your TA?
Your preview ends here
Eager to read complete document? Join bartleby learn and gain access to the full version
- Access to all documents
- Unlimited textbook solutions
- 24/7 expert homework help
BIOL 368 Lab Manual Week 4 19 Week 4 (home) Project 3: An Introduction to Microbial Genetics
A. Bacterial genetics
In this laboratory, you will be introduced to techniques for handling bacteria and methods used to examine the phenotypes of bacteria. In this course, we use bacteria to illustrate various aspects of genetics. First, they are small, grow quickly and do not require a complicated growth medium. This allows us to provide you with large numbers of cells that can be examined for mutants or can be used in mating experiments. Secondly, bacteria are haploid. Thus, when a mutation alters the function of a gene, the effect is obvious. A dominant wild-type gene does not mask it. E.coli
is a Gram-negative rod-shaped bacteria that is used for most of the genetics and molecular biology experiments. E.coli
cells can mate and exchange genetic information (conjugation), or genes can be transferred from one strain to another by a bacteriophage vector (transduction). B. subtilis
is a Gram-positive, rod-shaped bacteria that is used as a model organism for the endospore formation. The techniques and theoretical aspects of the experiments we do are pertinent to the general area of microbial genetics and to a lesser extent the genetic analysis of higher organisms. The techniques of microbial genetics are applied to the study of such problems as gene structure and function, the organization of genes in an organism, the transfer of genetic information from parents to offspring, the alteration of genetic information with time (evolution), the conditions that lead to the alteration of genetic information, the effects of altering the genetic information on the biochemistry and physiology of the cell, and the effects of introducing new genetic information into the cell. The purpose of the work carried out by microbial genetics might be basic (that is, to understand how the organism lives) or applied (that is, to have the organism do something useful for humans). For example, we could use genetics to gain an understanding of the regulation of amino acid biosynthesis - a basic problem; or we could use genetic techniques to isolate strains of bacteria that overproduce an amino acid so that we could harvest the amino acid and sell it - an applied problem. Microbial geneticists can manipulate genes either in vivo
or in vitro
and often combine the two approaches. An in vivo
method is one that uses the living organism or a population of organisms, whereas an in vitro
method is one in which cell constituents are isolated and manipulated in a tube. In this course, we will use both in vivo
and in vitro
methods.
BIOL 368 Lab Manual Week 4 20 B. Wild-type E. coli
K12 Most E. coli
geneticists work with derivatives of a strain of E. coli
isolated in 1922. This strain is E. coli
K-12. It is capable of synthesizing all of its biochemical needs from phosphates, ammonia, sulphate, and glucose. It is also able to use a wide variety of different carbon and energy sources other than glucose. We grow E. coli
K12 cells either in liquid media or on semi-solid media containing agar as the solidifying agent. Different media contain different nutrients and may contain indicator chemicals or chemicals that are toxic to wild-type cells. In liquid media, the cells form a uniform suspension, and all cells are exposed to essentially the same environment. Liquid cultures are easily manipulated and provide us with large populations of cells (1-5 X 10
9
cells/ mL). Such cultures are used as the starting material for many genetic experiments and are used for chemical and biochemical analyses of the cells. However, a liquid culture is a population of cells. Thus, it is difficult to look at the behaviour of single cells (or progeny of a single cell) in such a culture. To look at single cells (or more correctly, the progeny of single cells), we use semi-solid media. These are the same media we use for liquid cultures but have agar added. If we place a small number of bacterial cells on such a medium, the cells are not able to move from where they land and so divide and multiply to form macroscopic (visible to the naked eye) colonies. We use semi-solid media to isolate progeny of single cells and to enumerate cells. C. Mutants Over the years, thousands of mutant derivatives of E. coli
K-12 have been isolated from populations of cells using various strategies to differentiate between mutant and wild-type cells. More recently, DNA can be manipulated in vitro
to construct mutated genes, and these mutated genes can then be introduced into cells to replace the wild-type genes. Mutations can be moved from one strain to another using various genetic techniques. Thus strains can be isolated that have one or more genes altered. These strains have genotypes and phenotypes that are different from the wild-type strain. Recall that the genotype is the actual gene makeup of the organism, whereas the phenotype refers to the appearance or behaviour of the organism. It is easy to envisage the phenotype of a mutant fruit fly that has white eyes instead of red eyes, or of a pea plant that makes wrinkled peas rather than smooth peas, but it is more difficult to envisage mutant bacteria. What kinds of phenotypic changes can we observe in bacteria? i. Colony morphology mutants:
Bacterial colonies formed on semi-solid media have a characteristic morphology and colour. Mutants can have a different shape or texture or can be a different colour (so can contaminants). These mutants are relatively easy to isolate, as it is just a matter of looking at enough colonies to find odd-looking ones. However, they are not easy to work with genetically as there are no selections for or against them. Mutations giving rise to changes in colony morphology can affect different biochemical pathways. ii. Resistance mutants:
Mutant strains that are more resistant to toxic compounds than the wild-
type cells are relatively easy to isolate and to work with because cells with the mutant phenotype
BIOL 368 Lab Manual Week 4 21 can be readily selected by plating on a medium containing the compound. The biochemical change depends on the mode of action of the toxic compound, and so is different for each class of compounds. iii. Auxotrophic mutants:
These are mutants that require a compound that the wild-type makes for itself. For example, wild-type E. coli
grows on a minimal synthetic medium. This medium contains potassium phosphate, ammonium sulphate, sodium citrate, magnesium sulphate and glucose (or another carbon source). Other nutrients can be added if desired. For example, we can add the amino acid arginine. We can (with some difficulty) isolate mutants, which grow on the supplemented minimal medium but do not grow on the unsupplemented medium. These mutants are autotrophy mutants. Auxotrophic mutants generally are unable to carry out a particular step in the biosynthetic pathway of the required compound. These mutants were particularly useful in the elucidation of many biosynthetic pathways. Such mutants are difficult to isolate because you have to screen (see part D) a large number of colonies for the inability to grow on unsupplemented minimal medium (in contrast, resistant mutants can be selected by simply plating large numbers of cells on semi-solid medium containing the toxic chemical). Auxotrophs are reasonably easy to work with genetically as we can select wild-type revertants or recombinants from mutant populations. (How would you select for revertants of an arginine requiring auxotroph?) iv. Fermentation mutants:
E. coli
ferments a number of sugars with the production of organic acids as waste products. As a result, cells can use a fermentable sugar as the sole carbon source. Mutants, which cannot ferment the sugar, cannot grow on it. We can take advantage of various indicator media, which allow both wild-type and mutant strains to grow. However, these media contain a pH indicator that allows us to differentiate between mutant and wild-type colonies. Mutants unable to ferment a particular sugar are defective in the uptake of that sugar or in one of the steps in its degradation. Mutants unable to ferment or utilize a number of sugars are defective in common steps in the catabolism of the sugars. v. Temperature-sensitive mutants:
These conditionally lethal mutants grow at one temperature but do not grow at another. This type of mutant is useful since it enables us to isolate mutations affecting essential genes such as those involved in DNA replication. The change in the gene product is such that the protein or RNA is active at the lower temperature and not active at a higher temperature. Alternatively, the synthesis of the product might be inhibited at a higher temperature. Similarly, cold or pH-sensitive mutants can be isolated. Temperature-sensitive mutants are difficult to isolate but relatively easy to work with once you have them. vi. Suppressor mutants:
A suppressor mutation affects the expression of other mutations. For example, if you plate a large number of cells from an auxotrophic mutant that requires arginine on minimal agar, you would find that a few colonies are formed even though the majority of the cells are unable to reproduce. These colonies are now phenotypically wild-type and are called revertants. Some revertants are true revertants in which the exact wild-type genotype has been restored. Others are pseudo-revertants in which the mutation that restores the wild-type phenotype changes a different site on the chromosome than the original mutation. Pseudo-
Your preview ends here
Eager to read complete document? Join bartleby learn and gain access to the full version
- Access to all documents
- Unlimited textbook solutions
- 24/7 expert homework help
BIOL 368 Lab Manual Week 4 22 revertants contain two mutations, the original one that made the cells auxotrophic and the second one, which suppresses the first. The suppressor mutation can be within the same gene (intragenic suppressor) or in another gene (intergenic suppressor). It can also be gene-specific or allele-specific. In the first case, the suppressor suppresses any mutation affecting a specific gene, whereas in the second case, it will suppress mutations affecting a variety of genes but only some of the mutations. The mechanism of suppression depends on the nature of the suppressor mutation. One type of allele-specific intergenic suppressor results when a transfer RNA gene is mutated so that the tRNA decodes a codon it does not normally read. For example, amber suppressors result from tRNA mutations that produce a tRNA capable of reading the nonsense or stop codon UAG. vii. Brute force mutant screen:
Sometimes, biochemical analysis of the cell reveals a novel enzyme activity, but we are unable to find mutant cells when we look for phenotypic changes at the level of growth. Large numbers of individual cultures can be screened for the lack of biochemical activity. One classic example of such brute force isolation was the first polA
mutant. Early work had shown that E. coli
cells contain a DNA polymerase activity (that is, that synthesized DNA in vitro
). Also, a large number of temperature-sensitive mutants that were unable to synthesize DNA in vivo
when incubated at the non-permissive temperature were identified. When these mutants were screened for DNA polymerase activity, they all contained the activity. DeLucia and Cairns (Nature 224: 1164 (1969)) assayed about 2000 cultures derived from colonies of mutagenized E. coli
. They found one that lacked DNA polymerase activity. Surprisingly this mutant made DNA normally, although it was not able to repair DNA as well as the wild-type. These results indicated that the DNA polymerase activity that they were looking at was not crucial for DNA replication in the cell, and led to the discovery of two other DNA polymerase activities in E. coli
. D. Selection or screening? i. Selection
(noun) or select
(verb) describes the ability to define conditions where one type of cell present in a population grows whereas the other type does not grow. For example, a wild-
type population of E. coli
cells contains about 10 -10 streptomycin-resistant mutants. If we plate 1-5 x 10
10
cells on a plate containing streptomycin, there will be a few colonies on the plate after incubation. If we plate the same number of cells on the same medium without streptomycin, there will be no separate colonies. Instead, we observe a lawn of bacterial cells. ii. Screen
(noun or verb) describes conditions where individual colonies have to be examined for their phenotypes either by examining them by eye or by testing for growth or other properties. We have to screen for colony morphology mutants. E. The molecular basis of genotype and phenotype All organisms contain a large number of genes in the genome. The structure and function (that is, the phenotype) of a cell is determined by the pattern of gene expression (that is, the
Your preview ends here
Eager to read complete document? Join bartleby learn and gain access to the full version
- Access to all documents
- Unlimited textbook solutions
- 24/7 expert homework help
BIOL 368 Lab Manual Week 4 23 variety and amounts of different gene products) in the cell. The phenotype of a multicellular organism is determined by the combined phenotypes of cells expressing different sets of genes. A change in the amount or the activity of a gene product can result in a change in the phenotype of the organism. A gene product can be either RNA or protein. A sequence of deoxyribonucleotides containing one or more genes is transcribed into a sequence of ribonucleotides to give an RNA transcript. A transcript may be further processed to give the final gene product or a messenger RNA. A messenger RNA is translated into the amino acid sequence of a protein. Some portions of the DNA sequence do not encode gene products but are involved in the regulation of gene expression. Thus, a change in the DNA sequence can alter the function of a gene product or the expression of one or more genes. Such a mutation may alter the phenotype of the organism. F. Nomenclature Geneticists use conventions to describe the phenotype and genotype of different strains. The conventions vary for different groups of organisms. For example, the conventions used with mice are different from those used with fruit flies. The conventions for E. coli
and other bacteria are described here. Genotype
In general, genotypes are described by the 3-letter gene symbol in italics
. The symbol is based on the function of the gene when it is known, on the phenotype of mutants where the mutation affects a gene of unknown function, or on putative coding regions determined from the genome sequence. Gene symbols are changed to be more accurate when we learn the function of the gene. The gene symbol for an arginine biosynthetic gene is arg
. Similarly, the symbols for other amino acid biosynthetic genes are the three-letter abbreviations for the amino acids. You are expected to recognize the symbols for amino acid biosynthetic genes.
The symbol is written in lower case letters that are italicized. The symbol may be followed by a capital letter indicating a specific gene
(if the precise gene is known) and/or numbers indicating alleles
(different mutants). Other information is also given in the allele designation: ::
is used to indicate an insertion
Δ (delta) is used to indicate a deletion
Ω
(omega) is used to designate a fusion gene
(that is, a gene that is composed of part of one gene fused to part of another gene). o
arg-101
is an auxotrophic mutation that affects a gene involved in arginine biosynthesis. It is not known which gene is affected. The number designates allele identification.
Your preview ends here
Eager to read complete document? Join bartleby learn and gain access to the full version
- Access to all documents
- Unlimited textbook solutions
- 24/7 expert homework help
BIOL 368 Lab Manual Week 4 24 o
argA13
is an auxotrophic mutation where the argA
gene is known to be affected o
argA94::Tn10
is an auxotrophic mutation affecting the argA
gene. The mutation is caused by the insertion of the transposon Tn10 in the argA
gene. Transposon Tn10 also carries the tetracycline resistance gene, and therefore this strain is tetracycline resistant. Phenotype
The same 3-letter symbols are used to describe the phenotype but are written in regular font (not italic). Gene and allele designations are usually omitted. A superscript +
is used to denote the wild-type phenotype, whereas a -
is used to denote the mutant phenotype. Other superscripts may be used (for example, R for resistant and S for sensitive). Thus, strains containing either the arg-101
or the argA13
mutations are Arg
-
and the strains with no arg
mutations are Arg
+
. Strains are also given a name that normally consists of letters and numbers, which may or may not be assigned rationally. A complete strain description includes the strain name and its genotype (usually in parentheses). The description of the genotype lists only those genes that are known to be mutated. For example, strain MH458 (
supE thi-1 Δ
(lac-proAB) recA::Tn10 F’[traD36 proAB+ lacIq lacZΔM15] is a strain that was constructed in M. Herrington’
s laboratory. It is an amber suppressor (
supE
), requires the vitamin thiamine (
thi-1
), has a deletion of the lac-pro
region of the chromosome (
Δ
(lac-proAB)
), and is recombination deficient (
recA::Tn10
). It also contains the F’ episome or extrachromosomal element that carries a piece of the bacterial chromosome. The F factor portion of the episome contains a mutation in the traD
gene. This gene is required for the transfer of the F factor in conjugation, and so this strain does not transfer the F’ efficiently. The chromosomal portion of the F’ is a segment of DNA containing the lac-proAB
region and contains two lac
mutations. The lacI
q
mutation is a mutation in the gene (
lacI
) that codes for the repressor and results in the overproduction of the lac
repressor. The lacZΔM15
mutation is a deletion of part of the lacZ
gene. In addition to requiring thiamine, this strain will not grow on lactose as the sole carbon source. It is also tetracycline-resistant because of the Tn10. The best way to find information on a specific mutant allele, including genetic locations and mutant phenotype, is to consult a public database online. Below is a list of resources you will need to consult in this course. The Coli Genetic Stock Center http://cgsc.biology.yale.edu This site lists all E. coli
strains that are publically available. E. coli
genotypes at OpenWetWare http://openwetware.org/wiki/E._coli_genotypes This site lists only the E. coli
strains that are commonly used in molecular biology labs.
Your preview ends here
Eager to read complete document? Join bartleby learn and gain access to the full version
- Access to all documents
- Unlimited textbook solutions
- 24/7 expert homework help
BIOL 368 Lab Manual Week 4 25 H. The lac
Operon E. coli
can use many different carbon sources, including glucose and lactose. In order to use the disaccharide lactose, it must break it down into two monosaccharides: glucose and galactose. This reaction is catalyzed by the enzyme ß-galactosidase. ß-galactosidase is an inducible enzyme. That is, E. coli
normally does not make the enzyme unless it is given lactose as a carbon source. Moreover, it will only make ß-galactosidase if there is no glucose available. The study of these phenomena led to our earliest understanding of gene regulation. The lacZ
gene codes for the ß-galactosidase enzyme, which is part of an operon composed of three genes. The lacY
gene codes for lactose permease, a protein required for the uptake of lactose into the cell. lacA,
the third gene of the operon, codes for lactose transacetylase. The function of this enzyme is at this time still not known. Transcription of the lac
operon is controlled in two ways. Transcription is prevented (negative control) by the lac repressor (shown in the figure below) and is activated (positive control) by the cyclic AMP (cAMP) binding protein (CAP). The lac repressor, a protein encoded by the lacI
gene, prevents transcription by binding to the operator region of the operon. The repressor exists in two states. In the absence of the inducer, it binds specifically to the DNA sequence of the lac
operator. This binding prevents transcription of the operon. When lactose is present, it is converted to allolactose. This binds to the lac repressor and converts it to a form that cannot bind with a high affinity to the operator. IPTG, a synthetic inducer of the lac
operon, is often used in place of lactose in molecular biology experiments. The lac
operon then has the potential of being transcribed. However, it will be efficiently transcribed if CAP can bind to the CAP binding sites in the promoter region of the operon. CAP, like the lac repressor, can exist in two forms. In the absence of cAMP, it cannot bind efficiently to the CAP binding site. When cAMP is present, it binds to CAP, which then can bind to the CAP binding site. When glucose is available, the amount of cAMP in the cell is very low, and thus CAP does not activate transcription of the lac
operon. This is the way that the cell prevents the synthesis of ß-galactosidase when it has glucose available. The amount of cAMP increases when glucose is not available, and this allows CAP to activate transcription of the lac
operon. Elucidation of the structure and function of the lac
operon in the 1950s provided the first information on how gene expression is regulated. The work resulted in a Nobel Prize for Jacob
Your preview ends here
Eager to read complete document? Join bartleby learn and gain access to the full version
- Access to all documents
- Unlimited textbook solutions
- 24/7 expert homework help
BIOL 368 Lab Manual Week 4 26 and Monod in 1966. The lacZ
gene is now used extensively as an indicator gene in molecular biology. Its utility is based on the fact that ß-galactosidase activity is extremely easy to assay. In addition to cleaving lactose, ß-galactosidase is active on a number of artificial substrates, some of which are used in the laboratory for determining enzyme activity. The two most commonly used chromogenic substrates are ONPG (o-nitrophenyl-ß-D-galactopyranoside) and X-gal (5-bromo-4-chloro-3-indolyl-ß-D-galacto-
pyranoside). ONPG is used for quantitative enzyme assays, whereas X-gal is used for screening the Lac+ colonies on plates. When ONPG is split by ß-galactosidase, it yields a bright yellow product o-nitrophenol. The amount of o-nitrophenol can be determined using a spectrophotometer.
Your preview ends here
Eager to read complete document? Join bartleby learn and gain access to the full version
- Access to all documents
- Unlimited textbook solutions
- 24/7 expert homework help
BIOL 368 Lab Manual Week 4 27 When X-gal is split by ß-galactosidase, it yields a bright blue product (5,5’-dibromo-4,4'-dichloro-
indigo, compound 2 in the figure below) that colours the bacterial colony blue. Since neither X-gal nor ONPG is an inducer of the lac operon, artificial inducer Isopropyl β
-D-1-
thiogalactopyranoside (IPTG) must be added to the culture.
Your preview ends here
Eager to read complete document? Join bartleby learn and gain access to the full version
- Access to all documents
- Unlimited textbook solutions
- 24/7 expert homework help
BIOL 368 Lab Manual Week 4 28 Week 4 at-home assignment Preparation for Week 5 experiment: Testing mutant phenotypes
When you work with mutant strains of bacteria, it is important to check their phenotypes periodically to ensure that the strain is what you think it is. This is particularly important after obtaining a strain from another researcher, or after taking a strain out of storage. For this goal, you should be able to predict the phenotypes of each strain based on the genotype information. In Week 5 (next week), you’ll be given plates containing a wild-type strain (CAG12033) and seven different bacterial strains labelled as unknown 1-7. We know the identity of the seven strains, but we don’t know which one is which. Your task will be to identify the unknown 1-7 based on their phenotypes. To this goal, you need to know how to predict bacterial phenotypes based on the genotypes. These are the genotypes of the seven strains: BW6165 (
ara-41 lacY xyl7 mtlA-2 argE86::Tn10
)
CAG8209 (
λ
-, leuB6 thi-1 gal-6 lac glnV44 aer-
3075::Tn10
) CAG12204 (
λ
-, relA1, spoT1, metB1, btuB3192::Tn10kan
) CAG18475 (
λ
-
, metC162::Tn10
, rph-1
) D10 (
metB1 relA1 spoT1 rna-10
) MH142 (
metB lac
) MH807 (
argE3 rna
) They are grown on the following six agar media plates (1)
Min: Minimal media with glucose and thiamine (2)
MinR: Minimal media with glucose, thiamine and arginine (R represents arginine) (3)
MinL: Minimal media with glucose, thiamine and leucine (L represents leucine) (4)
MinM: Minimal media with glucose, thiamine and methionine (M represents methionine) (5)
Tet: LB medium containing tetracycline (6)
MacConkey Lactose 1.
Obtain all five mutant allele information of CAG12204 from The Coli Genetic Stock Center https://cgsc.biology.yale.edu/index.php
. Use the strain query for this and check each mutation. You may need to check the gene information from the mutant information page. •
What are they? •
What are the gene products? •
What are the expected phenotypes? If the information is not available, indicate ‘phenotype information not available.’ •
Our lab experiments (i.e. six agar plates) are designed to identify which mutation(s)?
Your preview ends here
Eager to read complete document? Join bartleby learn and gain access to the full version
- Access to all documents
- Unlimited textbook solutions
- 24/7 expert homework help
BIOL 368 Lab Manual Week 4 29 Present the information in a table format. 2.
Predict the growth (+ or -) and colony colour (only for MacConkey lactose media) of all seven strains and the wild-type strain. Present the information in a table format. If you are not sure what gene symbols mean, use the mutant query at The Coli Genetic Stock Center https://cgsc.biology.yale.edu/index.php
. 3.
Out of the seven strains, two of them are indistinguishable from the observations on these plates. Which ones? What type of experiments should you perform to distinguish the two? 4.
Add the reference list. Follow the proper citation format.
Your preview ends here
Eager to read complete document? Join bartleby learn and gain access to the full version
- Access to all documents
- Unlimited textbook solutions
- 24/7 expert homework help
BIOL 368 Lab Manual Week 5 30 Week 5 (lab) Project 3: An Introduction to Microbial Genetics (continued)
WARNING: Many students find the enzyme assay procedure to be very confusing. It is highly recommended to make good prelab and good mental preparation. Prelab should include a flow chart or drawing of procedures. Take time to watch the β
-galactosidase assay demonstration video. Watch the following demonstration videos before coming to the lab. Serological pipettes β
-galactosidase assay (30 min) Watch after making the prelab of the β
-galactosidase assay. You may have to add additional notes to the prelab after watching the video. You may have to watch twice or more to catch the detail. Bring a copy of the phenotype prediction table you make for Week 4 at-home assignment. You can either printout the assignment or copy the prediction into your lab book. Procedures A.
Demonstration: Phenotypes of wild-type and mutant of E. coli
Enter observations in the datasheet (done anytime before leaving the lab) A variety of mutant strains of E. coli
have been plated under conditions where you can compare their phenotypes to those of wild-type E. coli
. Examine the plates on the side bench and note the difference between the mutant strains and the wild-type strain. Please do not remove the lids from these plates. I.
Auxotrophic mutants
Strain JF1754 requires methionine, histidine, and leucine. It has been plated on four plates: minimal medium glucose (min) with methionine (M) and histidine (H); min with leucine (L) and M; min with H and L; and min with M, H and L. How does strain JF1754 compare to the wild-type strain, CAG12033? Why does strain JF1754 require the three amino acids to grow? II.
Antibiotic resistance
Strain EC5827 is resistant to streptomycin. Compare its growth on nutrient agar containing streptomycin with that of the wild-type strain, CAG12033.
Your preview ends here
Eager to read complete document? Join bartleby learn and gain access to the full version
- Access to all documents
- Unlimited textbook solutions
- 24/7 expert homework help
BIOL 368 Lab Manual Week 5 31 III.
The many colours of lac
The lac
operon is deleted from the chromosome of strain NK6042. Observe the plates containing strains NK6042 and CAG12033. IV.
min + lactose The minimal media normally contains glucose as the carbon source for bacterial growth but the glucose can be replaced with other compounds. Wild-type E. coli
can use a variety of compounds as carbon sources, including lactose. What is the phenotype of strain NK6042 on min + lactose? Compare it to the wild-type strain CAG12033. V.
MacConkey lactose This medium contains lactose and a complex carbon source (peptone, a meat product that contains amino acids and peptides). Thus both Lac
-
and Lac
+
cells can grow on this medium. It also contains a pH indicator that turns pink under acidic conditions. Lac
+
cells ferment lactose and excrete a mixture of organic acids, which causes the pH in the vicinity of the cells to decrease. Lac
-
cells do not acidify the medium. What colour are the strain NK6042 colonies? What colour are the CAG12033 colonies? VI.
X-gal plates X-gal is a colourless analog of lactose. Lac
+
strains will break it down, producing an insoluble blue compound. Lac
-
strains cannot break it down. On minimal plates containing glucose, Lac
+
strains make very little ß-galactosidase (the lacZ
product). They must be induced by lactose or another inducer to make the maximum amount of ß-galactosidase. Strains NK6042 and CAG12033 were streaked on plates of minimal medium containing glucose and X-gal (M-X plates) and minimal medium containing glucose, X-gal and an inducer of ß-galactosidase, isopropyl-ß-D-galactoside (IPTG). Compare the colours of the two strains. B. ß-galactosidase assay
Introduction: In demonstration plates, you observe several plate assays that allow you to detect whether E. coli
is making ß-galactosidase or not. The phenotype of a strain can also be monitored by using a biochemical assay for a gene product or the activity of a gene product. In this experiment, you will measure the activity of ß-galactosidase in different strains with and without the inducer. The chromogenic substrate o
-nitrophenyl-B-D-galactoside (ONPG) is used for the quantitative ß-
galactosidase activity. ß-galactosidase breaks ONPG into o
-nitrophenol, which is yellow, and galactose. The enzyme activity is quantified by yellow colour development (OD
420
). The strains you will test are: E. coli
strains CAG12033 (wild-type), MH321 (
metB1
lacZU118
), and ML308 (
lacI
). The three strains were grown overnight in minimal medium containing glucose and
Your preview ends here
Eager to read complete document? Join bartleby learn and gain access to the full version
- Access to all documents
- Unlimited textbook solutions
- 24/7 expert homework help
BIOL 368 Lab Manual Week 5 32 methionine, and in the same medium with added IPTG. IPTG induces the lac
operon even in the presence of glucose. Prelab assignment: 1. Create a flowchart depicting the procedures for the β
-galactosidase enzyme assay. 2. Based on the genotype of each strain, determine anticipated activities of β
-galactosidase. This information is used to predict the duration of the enzyme incubation. The high activity reactions must be stopped after a few minutes, whereas the low activity reactions can be incubated for up to two hours. Table 3.1. β
-galactosidase activity prediction Strain IPTG Anticipated activity (high, low or none) Enzyme assay tube ID CAG12033 - 1, 2 CAG12033 + 3, 4 MH321 - 5, 6 MH321 + 7, 8 ML308 - 9, 10 ML308 + 11, 12 Neg. Control (minA) Not applicable None 13 Blank (minA) Not applicable None 14 REMINDER: Record all data into your lab book. No data will be uploaded! Procedure
(
No need for aseptic techniques.) < dilution of bacterial cultures> 1.
You’ll be given six cultures. Dilute the cultures 10-fold (500 µL in 4.5 mL 1xMinA) in tall glass test tubes. The diluted cultures are used for i) OD
600
measurement and ii) β
-galactosidase enzyme assay. < β
-galactosidase enzyme assay> 1.
Label short glass test tubes 1-14. 2.
Put 1.8 mL of Z buffer (10 mM KCl, 1 mM MgSO
4
in 100 mM sodium phosphate, pH 7.0) in the
Your preview ends here
Eager to read complete document? Join bartleby learn and gain access to the full version
- Access to all documents
- Unlimited textbook solutions
- 24/7 expert homework help
BIOL 368 Lab Manual Week 5 33 tubes. 3.
Aliquot 200 µL of the diluted culture into the tubes, according to Table 3.1. For example, the diluted culture of CAG12033 grown without IPTG should be aliquoted to Tubes 1 and 2. Use MinA for Tube 13 & 14. *DO NOT discard the diluted cultures. You need them for the OD
600
measurement.* 4.
Put Tube 14 aside. This will be used as the blank for OD
420
and OD
550
. 5.
Label L for low or no activity tubes and H for high activity tubes. The control tube (Tube 13) should be L. There should be six H tubes and seven L tubes. 6.
Add four drops of chloroform, in the fume hood, and two drops of 0.2 % sodium dodecyl sulphate (SDS). Mix well by vortexing for 1 min. Vortexing can be done outside of the fume hood. This treatment opens cells, and proteins are now released to the solution. If your cells are not adequately permeabilized, you will have variable results. 7.
Place the tubes 1-13 & the tube containing ONPG in the 32
°
C water bath and allow them to warm up (approximately 5 minutes). Mix tubes periodically. 8.
Start reaction with low or no activity tubes. 9.
(Low and no activity tubes) Record the starting time. For these tubes, it is not necessary to record time separately. Add 400 µL of ONPG (4 mg/mL) to the low activity tubes (L label). Keep the samples in the 32
°
C water bath while adding substrate. Shake the tube for a few seconds. These tubes should be incubated for up to 2 hours. At the end of incubation, stop the reaction by adding 1 mL of 1 M sodium carbonate. Sodium carbonate stops reaction by quickly raising pH. You may not see the yellow colour from these tubes. That’s to be expected. Record the time when you add the sodium carbonate. The incubation time does not have to be exactly two hours. But you need to record the incubation time for calculation of enzyme activities. 10.
(High activity tubes) You should use a stopwatch for this step. You need to do the incubation one tube at a time. Have the stopwatch ready and start it when you add ONPG to the tube. Add 400 µL of ONPG (4 mg/mL) to the first high activity tubes (H label). Keep the samples in the 32
°
C water bath while adding substrate. Shake the tube for a few seconds. Incubate tubes at 32 o
C. Check the tubes periodically for yellow colour development due to the release of o-nitrophenol. Tubes should turn yellow in a few minutes. Stop the reaction by adding 1 mL of 1 M sodium carbonate. Sodium carbonate also enhances the yellow colour. Stop the stopwatch and record the time in your lab book.
Your preview ends here
Eager to read complete document? Join bartleby learn and gain access to the full version
- Access to all documents
- Unlimited textbook solutions
- 24/7 expert homework help
BIOL 368 Lab Manual Week 5 34 Repeat this for all H label tubes. Tubes can be kept at room temperature once the reaction is stopped. 11.
When the reactions have been stopped, measure OD
420
and OD
550
. You can measure OD
420
and OD
550 of high reaction tubes while you wait for low reaction tube incubations. Use Tube 14 as the blank. Zero the spectrophotometer at 420 nm before measuring OD
420
of samples. Zero the spectrophotometer at 550 nm again, then read OD
550
of the samples. OD
420
measures any yellow pigments, including cell debris. OD
550
is used to correct for the contribution made by the cell debris. The amount of o-nitrophenol is estimated by subtracting the OD
420
value due to the cell debris from the raw OD
420
reading. <OD
600
measurement>
1.
While you are waiting for the yellow colour to develop for low activity reactions, measure OD
600
of the diluted cell cultures, using MinA as the blank. <Enzyme activity calculation>
1.
Calculate the units of ß-galactosidase in each sample using the following equation: OD
420
-1.75
x OD
550
Miller unit
= 1000
x ---------------------------- t
x v
x
OD
600
OD
420
detects ο
-nitrophenol and cell debris OD
550
detects only cell debris 1.75 x OD
550
estimates
yellow colour due to cell debris
OD
600
:
cell density t
is the time of incubation in minutes. v
is the volume in mL. of culture assayed. It should be 0.2 for all calculations. v x OD
600
:
estimates the number of cells used for enzyme assay If you have difficulty with your calculation of enzyme assays, ask your TA for help. 2.
When doing your calculations, pay attention to the data. Do incubation times vary among different high reactions? Are any of your measured absorbance too high to be within the linear range of the assay? How do the OD
550
values vary? Looking at your data in this way helps you to develop the ability to look at raw data and roughly estimate what the results are, and whether they will be reasonably accurate or not. 3.
You may end up getting negative values for the low activity reactions. This means that the activity was below the detection limit. Instead of entering a negative value as enzyme activity, indicate ‘not detected.’
Your preview ends here
Eager to read complete document? Join bartleby learn and gain access to the full version
- Access to all documents
- Unlimited textbook solutions
- 24/7 expert homework help
BIOL 368 Lab Manual Week 5 35 Analysis of results: 1.
Are your calculated units for the induced wild-type strain within the expected range (approximately 1000-3000 units)? If not, have you made a calculation error or have you made a major experimental error? 2.
Are your results for the other assays what you predict from the strain descriptions? Briefly justify your answer. Did your duplicate samples agree within 20%? If not, what are the likely causes? 3.
In demonstration plates, you saw other ways of testing the Lac phenotype. If you were to test the three strains used here using other tests, what results would you expect? C. Testing the phenotypes of mutants You’ll be given seven different plates containing the following bacterial strains. BW6165 (
ara-41 lacY xyl7 mtlA-2 argE86::Tn10
)
CAG8209 (
λ
-, leuB6 thi-1 gal-6 lac glnV44 aer-
3075::Tn10
) CAG12204 (
λ
-, relA1, spoT1, metB1, btuB3192::Tn10kan
) CAG18475 (
λ
-
, metC162::Tn10
, rph-1
) D10 (
metB1 relA1 spoT1 rna-10
) MH142 (
metB lac
) MH807 (
argE3 rna
) You do not know which one is which, as the plates are labelled as Unknown 1, 2, etc. Your task is to identify them based on phenotype testing. They are grown on the following six agar media plates (1)
Min: Minimal media with glucose and thiamine (2)
MinR: Minimal media with glucose, thiamine and arginine (R represents arginine) (3)
MinL: Minimal media with glucose, thiamine and leucine (L represents leucine) (4)
MinM: Minimal media with glucose, thiamine and methionine (M represents methionine) (5)
Tet: LB medium containing tetracycline (6)
MacConkey Lactose Examine your plates. Record your results in a table by listing the media used and using a ‘+’ to indicate if a strain grew and a ‘-’ if it did not grow. Record the colony colour for MacConkey lactose media. Compare your results with the phenotype prediction you made in Week 4. Identify your unknowns.
Your preview ends here
Eager to read complete document? Join bartleby learn and gain access to the full version
- Access to all documents
- Unlimited textbook solutions
- 24/7 expert homework help
BIOL 368 Lab Manual Week 5 36 D.
Even bacteria can catch a virus Replication of bacteriophages (viruses which attack bacteria) occurs only within living bacteria. They are very specific in their activity. A bacteriophage for one strain of bacteria within a species may not attack another strain of the same species. This relationship is called bacteriophage to host specificity. The process by which phages infect cells (by injection of phage DNA) and the subsequent replication of phage particles and destruction of the cell with the release of virions is called the lytic cycle. If the lytic cycle occurs in broth culture, the turbidity of the culture decreases. If the lytic process occurs on an agar plate culture, plaques are formed. The plaque is a clear area produced by the destruction of the cells during successive cycles of phage replication on the lawn of bacteria (confluent growth host cells). Newly released phage attack adjacent bacterial host cells, destroy them and increase the size of the clearing or plaque. The number of phage particles in a given solution can be estimated by carrying out a serial dilution procedure. Each plaque formed would result from a single virus particle
. You will assay the number of plaque-forming units (PFU) in a sample of the Bacillus subtilis
phage SPO1. You will also study phage specificity by attempting to infect E. coli
with the SP01 phage. Read the instructions on the next page carefully before starting. The process is outlined in Figure 3-1. Figure 3-1:
Bacteriophage assay Step 1. Do a serial dilution of the phage in microfuge tubes. Use Tryptone broth as diluent.
Your preview ends here
Eager to read complete document? Join bartleby learn and gain access to the full version
- Access to all documents
- Unlimited textbook solutions
- 24/7 expert homework help
BIOL 368 Lab Manual Week 5 37 Step 2. Mix 0.1 mL of diluted phage with 0.1 mL of B. subtilis
(BD170) in sterile culture tubes. Step 3. Add 3 mL of soft nutrient agar (warm) to the mixture. Step 4. Immediately pour the soft agar mixture onto a nutrient agar plate and spread by swirling. Procedure:
COVID-19 note: Unfortunately, you won’t be doing the phage plating procedure. You will be given phage plates prepared by the technician. You will still need to see the following procedure to understand the dilution.
Use aseptic techniques.
1.
Take five sterile microfuge tubes; label them: 10
-1
, 10
-2
, 10
-3
, 10
-4
, and broth. Aseptically add 900 μL of nutrient broth in each tube.
2.
Prepare a ten-fold serial dilution of the phage as shown in Figure 3-1. NOTE: Remember to use a different sterile pipette tip for each transfer and mix the culture tube/dilutions before taking an aliquot and after dilutions were made. 3.
Obtain six sterile plastic culture tubes and 6 nutrient agar plates. Label them 1 to 6. 4.
Add bacteria and the phage dilution to the sterile tubes as specified in the table below. Test tube 1 2 3 4 5 6 Phage 100µL 10
-1
phage 100µL 10
-2
phage 100µL 10
-3
phage 100µL 10
-4
phage 100µL Broth 100µL 10
-1 phage Bacteria 100ųl
B. subtilis
100ųl
B. subtilis
100ųl
B. subtilis
100ųl
B. subtilis
100ųl B. subtilis
100ųl
E.coli
Your preview ends here
Eager to read complete document? Join bartleby learn and gain access to the full version
- Access to all documents
- Unlimited textbook solutions
- 24/7 expert homework help
BIOL 368 Lab Manual Week 5 38 5.
Once you are ready, move to an area closer to soft agar. A bottle of soft top agar is kept in the 55 o
C water bath. Bring the 6 sterile culture tubes, 6 nutrient agar plates and a sterile 5-mL pipette near the 55 o
C water bath area. (Do not perform the following procedures until you watched the demonstration.) 6.
Put the tubes you prepared in step 4 in the water bath. Pipette 3 mL of soft agar into the tubes. (No need to mix the tubes, as this may introduce bubbles in the agar.) Pour the contents of the soft agar onto a nutrient agar plate. Work quickly as agar can solidify in the tube. Gently swirl the agar plate to distribute the soft agar evenly over the surface. Avoid introducing bubbles. Keep the dish right side up until agar solidifies. 7.
Repeat step 6 until you have finished all the six tubes. 8.
Let the agar solidify for about 20 min. Place the agar plates upside down on the tray to be incubated at 37°C. 9.
Discard all the contaminated tubes in the biohazard waste. 10.
Plates will be put in the refrigerator the next day. Phage plate observation
1.
Phage plates were prepared by the technician, as described above. You’ll need to observe the phage plates. 2.
Count the number of plaques on each plate. Calculate the PFU/mL of the original phage solution. As with the viable counts, plaque counts of 30-300/plate give you the most accurate estimate of the titer. 3.
Record your results in your lab book & datasheet. Check other groups’ results. No plaque means ‘lawn of bacteria,’ and fused plaques mean ‘confluent lysis.’ 4.
Were there any plaques when E. coli
was used as the plating bacteria? How many PFU did you put on that plate? What possible explanations can you think of for the host specificity of a bacteriophage? 5.
Download the section data from Moodle. Calculate the phage titer (PFU/mL) for each group. Calculate section average and standard deviations. Compare section data against your data. End of experiments. Have you cleaned up your bench? Have you given your group data to your TA?
Your preview ends here
Eager to read complete document? Join bartleby learn and gain access to the full version
- Access to all documents
- Unlimited textbook solutions
- 24/7 expert homework help
Related Documents
Recommended textbooks for you
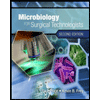
Microbiology for Surgical Technologists (MindTap ...
Biology
ISBN:9781111306663
Author:Margaret Rodriguez, Paul Price
Publisher:Cengage Learning
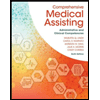
Comprehensive Medical Assisting: Administrative a...
Nursing
ISBN:9781305964792
Author:Wilburta Q. Lindh, Carol D. Tamparo, Barbara M. Dahl, Julie Morris, Cindy Correa
Publisher:Cengage Learning
Surgical Tech For Surgical Tech Pos Care
Health & Nutrition
ISBN:9781337648868
Author:Association
Publisher:Cengage
Essentials Health Info Management Principles/Prac...
Health & Nutrition
ISBN:9780357191651
Author:Bowie
Publisher:Cengage
Recommended textbooks for you
- Microbiology for Surgical Technologists (MindTap ...BiologyISBN:9781111306663Author:Margaret Rodriguez, Paul PricePublisher:Cengage LearningComprehensive Medical Assisting: Administrative a...NursingISBN:9781305964792Author:Wilburta Q. Lindh, Carol D. Tamparo, Barbara M. Dahl, Julie Morris, Cindy CorreaPublisher:Cengage Learning
- Surgical Tech For Surgical Tech Pos CareHealth & NutritionISBN:9781337648868Author:AssociationPublisher:CengageEssentials Health Info Management Principles/Prac...Health & NutritionISBN:9780357191651Author:BowiePublisher:Cengage
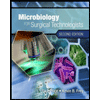
Microbiology for Surgical Technologists (MindTap ...
Biology
ISBN:9781111306663
Author:Margaret Rodriguez, Paul Price
Publisher:Cengage Learning
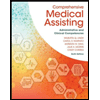
Comprehensive Medical Assisting: Administrative a...
Nursing
ISBN:9781305964792
Author:Wilburta Q. Lindh, Carol D. Tamparo, Barbara M. Dahl, Julie Morris, Cindy Correa
Publisher:Cengage Learning
Surgical Tech For Surgical Tech Pos Care
Health & Nutrition
ISBN:9781337648868
Author:Association
Publisher:Cengage
Essentials Health Info Management Principles/Prac...
Health & Nutrition
ISBN:9780357191651
Author:Bowie
Publisher:Cengage