SU_BIO2071_W6_A2_BAKER_A
docx
keyboard_arrow_up
School
South University, Savannah *
*We aren’t endorsed by this school
Course
2071
Subject
Biology
Date
Jan 9, 2024
Type
docx
Pages
6
Uploaded by EarlGoat3802
Your preview ends here
Eager to read complete document? Join bartleby learn and gain access to the full version
- Access to all documents
- Unlimited textbook solutions
- 24/7 expert homework help
Your preview ends here
Eager to read complete document? Join bartleby learn and gain access to the full version
- Access to all documents
- Unlimited textbook solutions
- 24/7 expert homework help
Recommended textbooks for you
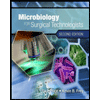
Microbiology for Surgical Technologists (MindTap ...
Biology
ISBN:9781111306663
Author:Margaret Rodriguez, Paul Price
Publisher:Cengage Learning
Surgical Tech For Surgical Tech Pos Care
Health & Nutrition
ISBN:9781337648868
Author:Association
Publisher:Cengage
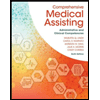
Comprehensive Medical Assisting: Administrative a...
Nursing
ISBN:9781305964792
Author:Wilburta Q. Lindh, Carol D. Tamparo, Barbara M. Dahl, Julie Morris, Cindy Correa
Publisher:Cengage Learning
Recommended textbooks for you
- Microbiology for Surgical Technologists (MindTap ...BiologyISBN:9781111306663Author:Margaret Rodriguez, Paul PricePublisher:Cengage Learning
- Surgical Tech For Surgical Tech Pos CareHealth & NutritionISBN:9781337648868Author:AssociationPublisher:CengageComprehensive Medical Assisting: Administrative a...NursingISBN:9781305964792Author:Wilburta Q. Lindh, Carol D. Tamparo, Barbara M. Dahl, Julie Morris, Cindy CorreaPublisher:Cengage Learning
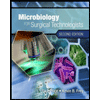
Microbiology for Surgical Technologists (MindTap ...
Biology
ISBN:9781111306663
Author:Margaret Rodriguez, Paul Price
Publisher:Cengage Learning
Surgical Tech For Surgical Tech Pos Care
Health & Nutrition
ISBN:9781337648868
Author:Association
Publisher:Cengage
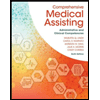
Comprehensive Medical Assisting: Administrative a...
Nursing
ISBN:9781305964792
Author:Wilburta Q. Lindh, Carol D. Tamparo, Barbara M. Dahl, Julie Morris, Cindy Correa
Publisher:Cengage Learning