SERVICE ET AL. WILEY 5 of 9 TABLE 1 Observed (O) versus expected (E) mc1r genotypes of black bears (Ursus americanus) by landmass in coastal British Columbia, Canada (2012-2017). Monte Carlo Exact Chi-square p-values reflect results from tests for departures from genotype frequencies expected under Hardy-Weinberg equilibrium. U-score p-values reflect tests for an excess or deficiency of heterozygosity at mc1r Landmass GG (white) AG (black) AA (black) о E ° E O E Chi² p U-score p Island Hawkesbury 0 0.00 0 0.00 24 24.00 Gribbell 1 1.13 7 6.75 10 10.12 1.00 0.74 Princess Royal 8 5.32 25 30.36 46 43.32 0.14 0.09 Roderick 0 0.01 1 0.98 21 21.01 $ 1.00 1.00 Pooley 0 0.00 0 0.00 6 6.00 - Yeo 0 0.00 0 0.00 5 5.00 Mainland West of Hawkesbury 0 0.03 East of Princess Royal 0 0.15 North of Roderick 0 0.02 Kynoch 0 0.00 Don Peninsula 0 0.01 24101 1.94 34 34.03 1.00 0.99 3.70 23 23.15 1.00 0.89 0.96 11 11.02 1.00 1.00 0.00 15 15.00 0.98 21 21.01 1.00 - 1.00 alleles. We fit a Matern variogram model (Cressie, 1990) using maxi- mum likelihood to account for spatial structure in G allele frequency values. The selected variogram model was used to fit a cost-based weighted kriging model using the krig.conv function in the geoRcb pack- age (López-Quílez & Muñoz, 2009) to create the interpolated G allele frequency surface. We used the predicted surface to identify hotspots of G allele frequencies and assess their spatial alignment with pro- tected areas (Section 2.5.3). 2.5.3 Assessing alignment of protected areas with G allele hotspots We assessed the alignment between our kriged G allele frequency surface and protected areas through two complementary approaches. First, we identified hotspots of allele frequency, defined as pixels with values in the 90th percentile (i.e. top 10% of the entire interpolated raster surface from Section 2.5.2) and assessed the percentage of the hotspot that corresponded with protected areas. To assess sensitivity of this arbitrary, but logical, cutoff value, we also report values for the 95th (top 5%) and 85th percentile (top 15%) hotspots. Secondly, we cal- culated the percentage of protected area in each landmass and tested if this value was predicted by G allele frequency through a linear regres- sion model and a Pearson's correlation. 3 RESULTS to 0.26 (Princess Royal Island), were mostly lower than previously reported estimates, and were significantly lower on Roderick (Odds ratio=0.091; p = 0.018) and Gribbell (Odds ratio = .261; p = 0.007) Islands (Table 2). Also, in contrast with previous research, we did not detect a heterozygote deficiency. Rather, we failed to detect a statis- tically significant departure from Hardy-Weinberg equilibrium in any landmass (Table 1; Table 2). We found that multiple regions of high G allele frequency occurred outside of protected areas. Specifically, approximately 50% of the 90th percentile hotspots corresponded with protected areas (Figure 2; Table 2). Similar results emerged for the 95th and 85th percentiles, at approximately 50% and approximately 45% protected area cover- age, respectively. Across landmasses, protected area coverage was not related spatially to the G allele frequency (R = -0.012; F = 0.001; p = 0.971). The two landmasses with the highest G allele frequency differed strongly in protected area coverage, with Princess Royal Island (G frequency = 0.26) having high coverage (52% of area) and Gribbell Island (G frequency = 0.25) having very low protection (0.05%; Table 2). 4 DISCUSSION Our results suggest that landscape-level frequency of the G allele is lower than previously estimated, and that populations previously reported to demonstrate a heterozygote deficiency are in fact in Hardy-Weinberg equilibrium. Additionally, despite the role of Spirit
SERVICE ET AL. WILEY 5 of 9 TABLE 1 Observed (O) versus expected (E) mc1r genotypes of black bears (Ursus americanus) by landmass in coastal British Columbia, Canada (2012-2017). Monte Carlo Exact Chi-square p-values reflect results from tests for departures from genotype frequencies expected under Hardy-Weinberg equilibrium. U-score p-values reflect tests for an excess or deficiency of heterozygosity at mc1r Landmass GG (white) AG (black) AA (black) о E ° E O E Chi² p U-score p Island Hawkesbury 0 0.00 0 0.00 24 24.00 Gribbell 1 1.13 7 6.75 10 10.12 1.00 0.74 Princess Royal 8 5.32 25 30.36 46 43.32 0.14 0.09 Roderick 0 0.01 1 0.98 21 21.01 $ 1.00 1.00 Pooley 0 0.00 0 0.00 6 6.00 - Yeo 0 0.00 0 0.00 5 5.00 Mainland West of Hawkesbury 0 0.03 East of Princess Royal 0 0.15 North of Roderick 0 0.02 Kynoch 0 0.00 Don Peninsula 0 0.01 24101 1.94 34 34.03 1.00 0.99 3.70 23 23.15 1.00 0.89 0.96 11 11.02 1.00 1.00 0.00 15 15.00 0.98 21 21.01 1.00 - 1.00 alleles. We fit a Matern variogram model (Cressie, 1990) using maxi- mum likelihood to account for spatial structure in G allele frequency values. The selected variogram model was used to fit a cost-based weighted kriging model using the krig.conv function in the geoRcb pack- age (López-Quílez & Muñoz, 2009) to create the interpolated G allele frequency surface. We used the predicted surface to identify hotspots of G allele frequencies and assess their spatial alignment with pro- tected areas (Section 2.5.3). 2.5.3 Assessing alignment of protected areas with G allele hotspots We assessed the alignment between our kriged G allele frequency surface and protected areas through two complementary approaches. First, we identified hotspots of allele frequency, defined as pixels with values in the 90th percentile (i.e. top 10% of the entire interpolated raster surface from Section 2.5.2) and assessed the percentage of the hotspot that corresponded with protected areas. To assess sensitivity of this arbitrary, but logical, cutoff value, we also report values for the 95th (top 5%) and 85th percentile (top 15%) hotspots. Secondly, we cal- culated the percentage of protected area in each landmass and tested if this value was predicted by G allele frequency through a linear regres- sion model and a Pearson's correlation. 3 RESULTS to 0.26 (Princess Royal Island), were mostly lower than previously reported estimates, and were significantly lower on Roderick (Odds ratio=0.091; p = 0.018) and Gribbell (Odds ratio = .261; p = 0.007) Islands (Table 2). Also, in contrast with previous research, we did not detect a heterozygote deficiency. Rather, we failed to detect a statis- tically significant departure from Hardy-Weinberg equilibrium in any landmass (Table 1; Table 2). We found that multiple regions of high G allele frequency occurred outside of protected areas. Specifically, approximately 50% of the 90th percentile hotspots corresponded with protected areas (Figure 2; Table 2). Similar results emerged for the 95th and 85th percentiles, at approximately 50% and approximately 45% protected area cover- age, respectively. Across landmasses, protected area coverage was not related spatially to the G allele frequency (R = -0.012; F = 0.001; p = 0.971). The two landmasses with the highest G allele frequency differed strongly in protected area coverage, with Princess Royal Island (G frequency = 0.26) having high coverage (52% of area) and Gribbell Island (G frequency = 0.25) having very low protection (0.05%; Table 2). 4 DISCUSSION Our results suggest that landscape-level frequency of the G allele is lower than previously estimated, and that populations previously reported to demonstrate a heterozygote deficiency are in fact in Hardy-Weinberg equilibrium. Additionally, despite the role of Spirit
Human Anatomy & Physiology (11th Edition)
11th Edition
ISBN:9780134580999
Author:Elaine N. Marieb, Katja N. Hoehn
Publisher:Elaine N. Marieb, Katja N. Hoehn
Chapter1: The Human Body: An Orientation
Section: Chapter Questions
Problem 1RQ: The correct sequence of levels forming the structural hierarchy is A. (a) organ, organ system,...
Related questions
Question
Consider all Islands with documented occurrences of the mc1r allele “G” as population 1 (the 3 Islands where “G” was detected). All mainland bears are population 2. Use the values in “Table 1” to calculate the allelic and genotypic frequencies for each population. calculate vertically ( (1+8+7+25+1+24+10+46+21+6+5)

Transcribed Image Text:SERVICE ET AL.
WILEY
5 of 9
TABLE 1 Observed (O) versus expected (E) mc1r genotypes of black bears (Ursus americanus) by landmass in coastal British Columbia, Canada
(2012-2017). Monte Carlo Exact Chi-square p-values reflect results from tests for departures from genotype frequencies expected under
Hardy-Weinberg equilibrium. U-score p-values reflect tests for an excess or deficiency of heterozygosity at mc1r
Landmass
GG (white)
AG (black)
AA (black)
о
E
°
E
O
E
Chi² p
U-score p
Island
Hawkesbury
0
0.00
0
0.00
24
24.00
Gribbell
1
1.13
7
6.75
10
10.12
1.00
0.74
Princess Royal
8
5.32
25
30.36
46
43.32
0.14
0.09
Roderick
0
0.01
1
0.98
21
21.01
$ 1.00
1.00
Pooley
0
0.00
0
0.00
6
6.00
-
Yeo
0
0.00
0
0.00
5
5.00
Mainland
West of Hawkesbury
0
0.03
East of Princess Royal
0
0.15
North of Roderick
0
0.02
Kynoch
0
0.00
Don Peninsula
0
0.01
24101
1.94
34
34.03
1.00
0.99
3.70
23
23.15
1.00
0.89
0.96
11
11.02
1.00
1.00
0.00
15
15.00
0.98
21
21.01
1.00
-
1.00
alleles. We fit a Matern variogram model (Cressie, 1990) using maxi-
mum likelihood to account for spatial structure in G allele frequency
values. The selected variogram model was used to fit a cost-based
weighted kriging model using the krig.conv function in the geoRcb pack-
age (López-Quílez & Muñoz, 2009) to create the interpolated G allele
frequency surface. We used the predicted surface to identify hotspots
of G allele frequencies and assess their spatial alignment with pro-
tected areas (Section 2.5.3).
2.5.3 Assessing alignment of protected areas with
G allele hotspots
We assessed the alignment between our kriged G allele frequency
surface and protected areas through two complementary approaches.
First, we identified hotspots of allele frequency, defined as pixels with
values in the 90th percentile (i.e. top 10% of the entire interpolated
raster surface from Section 2.5.2) and assessed the percentage of the
hotspot that corresponded with protected areas. To assess sensitivity
of this arbitrary, but logical, cutoff value, we also report values for the
95th (top 5%) and 85th percentile (top 15%) hotspots. Secondly, we cal-
culated the percentage of protected area in each landmass and tested if
this value was predicted by G allele frequency through a linear regres-
sion model and a Pearson's correlation.
3 RESULTS
to 0.26 (Princess Royal Island), were mostly lower than previously
reported estimates, and were significantly lower on Roderick (Odds
ratio=0.091; p = 0.018) and Gribbell (Odds ratio = .261; p = 0.007)
Islands (Table 2). Also, in contrast with previous research, we did not
detect a heterozygote deficiency. Rather, we failed to detect a statis-
tically significant departure from Hardy-Weinberg equilibrium in any
landmass (Table 1; Table 2).
We found that multiple regions of high G allele frequency occurred
outside of protected areas. Specifically, approximately 50% of the
90th percentile hotspots corresponded with protected areas (Figure 2;
Table 2). Similar results emerged for the 95th and 85th percentiles,
at approximately 50% and approximately 45% protected area cover-
age, respectively. Across landmasses, protected area coverage was not
related spatially to the G allele frequency (R = -0.012; F = 0.001;
p = 0.971). The two landmasses with the highest G allele frequency
differed strongly in protected area coverage, with Princess Royal
Island (G frequency = 0.26) having high coverage (52% of area) and
Gribbell Island (G frequency = 0.25) having very low protection (0.05%;
Table 2).
4 DISCUSSION
Our results suggest that landscape-level frequency of the G allele
is lower than previously estimated, and that populations previously
reported to demonstrate a heterozygote deficiency are in fact in
Hardy-Weinberg equilibrium. Additionally, despite the role of Spirit
Expert Solution

This question has been solved!
Explore an expertly crafted, step-by-step solution for a thorough understanding of key concepts.
This is a popular solution!
Trending now
This is a popular solution!
Step by step
Solved in 1 steps

Recommended textbooks for you
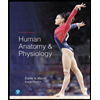
Human Anatomy & Physiology (11th Edition)
Biology
ISBN:
9780134580999
Author:
Elaine N. Marieb, Katja N. Hoehn
Publisher:
PEARSON
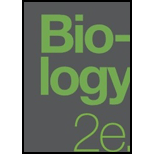
Biology 2e
Biology
ISBN:
9781947172517
Author:
Matthew Douglas, Jung Choi, Mary Ann Clark
Publisher:
OpenStax
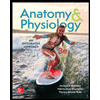
Anatomy & Physiology
Biology
ISBN:
9781259398629
Author:
McKinley, Michael P., O'loughlin, Valerie Dean, Bidle, Theresa Stouter
Publisher:
Mcgraw Hill Education,
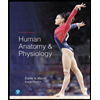
Human Anatomy & Physiology (11th Edition)
Biology
ISBN:
9780134580999
Author:
Elaine N. Marieb, Katja N. Hoehn
Publisher:
PEARSON
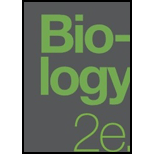
Biology 2e
Biology
ISBN:
9781947172517
Author:
Matthew Douglas, Jung Choi, Mary Ann Clark
Publisher:
OpenStax
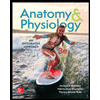
Anatomy & Physiology
Biology
ISBN:
9781259398629
Author:
McKinley, Michael P., O'loughlin, Valerie Dean, Bidle, Theresa Stouter
Publisher:
Mcgraw Hill Education,
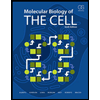
Molecular Biology of the Cell (Sixth Edition)
Biology
ISBN:
9780815344322
Author:
Bruce Alberts, Alexander D. Johnson, Julian Lewis, David Morgan, Martin Raff, Keith Roberts, Peter Walter
Publisher:
W. W. Norton & Company
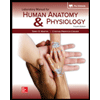
Laboratory Manual For Human Anatomy & Physiology
Biology
ISBN:
9781260159363
Author:
Martin, Terry R., Prentice-craver, Cynthia
Publisher:
McGraw-Hill Publishing Co.
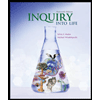
Inquiry Into Life (16th Edition)
Biology
ISBN:
9781260231700
Author:
Sylvia S. Mader, Michael Windelspecht
Publisher:
McGraw Hill Education