What is the Electron Transport Chain (ETC)?
The electron transport chain, also known as the electron transport system, is a group of proteins that transfer electrons through a membrane within mitochondria to create a gradient of protons that drives adenosine triphosphate (ATP)synthesis. The cell uses ATP as an energy source for metabolic processes and cellular functions. ETC involves series of reactions that convert redox energy from NADH (nicotinamide adenine dinucleotide (NAD) + hydrogen (H)) and FADH2(flavin adenine dinucleotide (FAD)) oxidation into proton-motive force(PMF), which is then used to synthesize ATP through conformational changes in the ATP synthase complex, a process known as oxidative phosphorylation.
Oxidative Phosphorylation
By reducing equivalent NADH, FADH2 generated during glycolysis, and the link between glycolysis and Krebs cycle, oxidative phosphorylation is used to synthesize ATP.
Oxidative phosphorylation involves two components:
- Electron transport chain
- ATP synthase
Proton motive force is generated by the flow of electrons from the reducing equivalence across the electron transport chain. The stored energy in PMF is used to power ATP synthesis. This PMF is used by ATP synthase to drive ATP synthesis.
During this stage, a series of electrons are transferred from organic compounds to oxygen while simultaneously releasing energy. The final electron acceptor in aerobic respiration is molecular oxygen, whereas, in anaerobic respiration, other acceptors such as sulphate are used.
The chain of reactions is significant because it involves the breakdown of ATP into ADP (adenosine diphosphate) and the subsequent resynthesize of ADP into ATP, utilizing the body's limited ATPs which is approximately 300 times per day.
Components of the Electron Transport Chain
The electron transport chain is made up of a series of asymmetrically arranged electron carriers in the membrane.
In bacteria, the membrane is the cytoplasmic membrane, while in eukaryotes, the membrane is the inner mitochondrial membrane.
The electron carriers are arranged in sequential order, and they are reduced as they accept electrons from previous carriers and oxidized as they pass electrons to subsequent carriers.
The different electron carriers are:
- NADH dehydrogenase
- Iron-sulfur (Fe) center
- Ubiquinone(UQ)
- Flavoproteins (flavin mononucleotide (FMN) and FAD)
- Cytochrome (cyt)
Electron transport is described as a series of redox reactions resembling a relay race. It is a type of aerobic breathing. It is the only part of the glucose metabolism process that uses oxygen from the atmosphere. When electrons pass from one component to the next until they reach the end of the chain, they reduce molecular oxygen, resulting in the production of water. The need for oxygen in the final phase can be seen in a chemical reaction that necessitates the use of both oxygen and glucose.
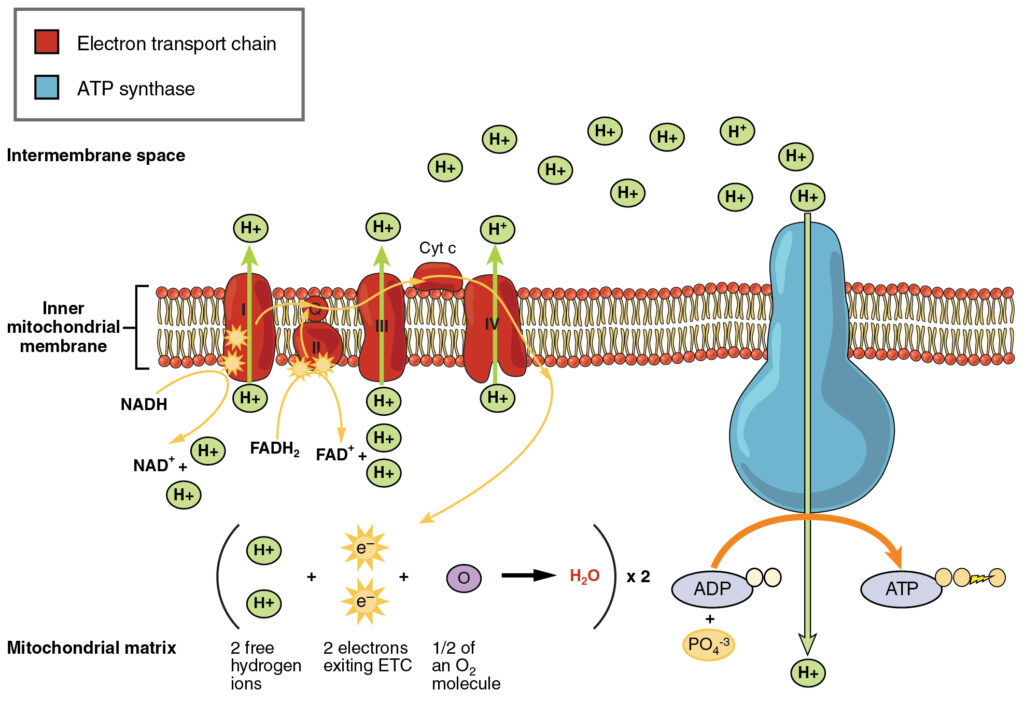
Electron Transport Chain Complexes
The respiratory chain, also known as the electron transport chain, is made up of four large protein complexes embedded in the inner mitochondrial membrane that transport electrons.
This stage is critical in energy synthesis because all oxidative steps in the degradation of carbohydrates, fats, and amino acids converge at this final stage of cellular respiration, where the energy of oxidation drives the synthesis of ATP.
Electron Transport Chain in Mitochondria
A complex is a structure made up of proteins, molecules, or atoms that are weakly connected to a large protein. Prokaryotes have multiple copies of the electron transport chain in their plasma membrane.
- Complex I: NADH-quinone oxidoreductase is made up of iron-sulfur and FMN-containing enzymes. Two electrons are carried to the first complex aboard NADH in this step. FMN is a vitamin B2 derivative.
- Q and complex II: Succinate-Q reductase: FADH2, that does not pass through complex I is received directly from complex II. Through the compound ubiquinone, the first and second complexes are linked to a third complex (Q). The Q molecule is water-soluble and moves freely in the membrane's hydrophobic core. An electron is delivered directly to the electron protein chain during this phase. The amount of ATP produced at this stage is dependent on the number of protons pumped across the inner membrane of the mitochondria.
- Complex III: The third complex, cytochrome c reductase, is made up of Fe-S protein, cytochrome b, and cytochrome c proteins. The heme group is found in cytochrome proteins. The job of complexity is to pump protons across the membrane. It also transports electrons to the fourth complex of enzymes and proteins through cytochrome c. The electron donor is Q, and the electron acceptor is cytochrome c.
- Complex IV: Cytochrome c oxidase: Cytochrome c, a, and a3 make up the fourth complex. In cytochromes c and a3, two heme groups are presenting each. The cytochromes are in charge of holding oxygen molecules between copper and iron until they are completely depleted of oxygen. Reduced oxygen picks up two hydrogen ions from the surrounding environment to form water in this phase.
Arrangement of the Five Electron Carriers
The five electron carriers arranged in the form of four complexes are as follows.
- Complex I: NADH quinone oxidoreductase complex (NADH to quinone)
NADH → FMN → FeS → Q
- Complex II: Succinate dehydrogenase complex (Succinate to quinone)
Succinate → FAD → FeS →Q
- Complex III: cytochrome bc1 (Ubiquinone to cytochrome c)
UQ2 → cyt b-c1 → cyt c
- Complex IV: Cytochrome oxidase (cytc to O2)
cyt c → cyt a → cyt a3 → O2
Electron Transport Chain Steps
The following steps are involved in the ETC:
- Transfer of electrons from NADH to ubiquinone
- The TCA (tricarboxylic acid) cycle's ketoglutarate dehydrogenase, isocitrate dehydrogenase, and malate dehydrogenase reactions; the pyruvate dehydrogenase reaction that converts pyruvate to acetyl CoA (coenzyme A); oxidation of fatty acids, and other oxidation reactions all produce NADH.
- The intermembrane space receives NADH produced in the mitochondrial matrix.
- The electrons are then transferred to FMN in the intermembrane space through the complex I(NADH dehydrogenase).
- The electrons are then transferred to the Fe-S center (one electron to one Fe-S center) by FMN, which then transfers the electrons to CoQ one by one, forming semiquinone and then ubiquinol.
- The energy generated by the electron transfer is used to pump two protons across the membrane, resulting in a potential gradient.
- The protons in the ATP synthase complex return to the matrix through the pore, forming energy in the form of ATP.
- Transfer of electrons from FADH2 to CoQ
- The reduction of FAD to FADH2 occurs when succinate is oxidized to fumarate.
- Complex II, succinic dehydrogenase, catalyzes the electron transport chain, which accepts electrons from FADH2.
- The electrons reach CoQ through a series of Fe-S centers, just as they did in complex I.
- Complex II, on the other hand, does not pump any protons across the membrane.
- Transfer of electrons from ubiquinol (CoQH2)tocytochrome c
- Electrons are transferred from reduced CoQH2 to cytochrome b and c1 and then to cytochrome c.
- This process is catalyzed by complex II (cytochrome reductase), which reduces Fe3+ in the cytochrome to Fe2+.
- Each cytochrome transfers one electron, resulting in the reduction of two cytochrome molecules for every NADH oxidized.
- The transfer of electrons generates energy, which is used to pump protons across the membrane, thereby assisting the potential gradient.
- The protons return to the matrix through the pore in the ATP synthase complex, where they generate energy in the form of ATP, just as they did in the first step.
- Transfer of electrons from cytochrome c to molecular oxygen
- Complex IV (cytochrome oxidase) catalyzes the final step in the electron transfer chain, where electrons are transferred from cytochrome c to molecular oxygen.
- Because it takes two electrons to reduce one molecule of oxygen to water, each NADH oxidation results in the reduction of half of the oxygen to water.
- The Fe2+ in cytochrome c is oxidized to Fe3+ in a similar way. Protons are pumped across the membrane using the energy released during the process.
- The formation of ATP is caused by the return of protons to the matrix.
Chemiosmosis
The free energy generated by the redox reactions just described is used to pump hydrogen ions (protons) across the membrane in chemiosmosis. Due to the positive charge of hydrogen ions and their aggregation on one side of the membrane, the uneven distribution of H+ ions across the membrane establishes both concentration and electrical gradients (thus, an electrochemical gradient).
The ions would tend to diffuse back across the membrane into the matrix if the membrane was open to the diffusion of H+ ions, driven by their electrochemical gradient. Many ions require the assistance of ion channels to diffuse through the nonpolar regions of phospholipid membranes. In the same way, hydrogen ions in the matrix space can only pass through the inner mitochondrial membrane through ATP synthase, an integral membrane protein. The force of the hydrogen ions diffusing through it, down their electrochemical gradient, turns this complex protein into a tiny generator. Using the potential energy from the hydrogen ion gradient, the conformational changes in the ATP synthase facilitate the addition of a phosphate to ADP, forming ATP.
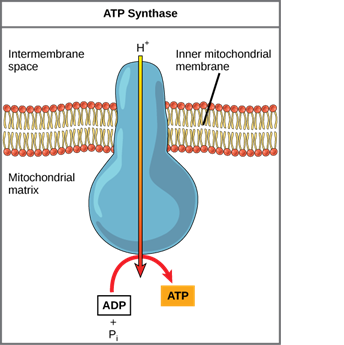
Chemiosmosis is a light-dependent method used during the light reactions of photosynthesis to harness the energy of sunlight through photophosphorylation. It is used to generate 90% of the ATP produced during aerobic glucose catabolism. Remember that oxidative phosphorylation is the process of producing ATP in mitochondria through chemiosmosis. The energy of the electrons removed from hydrogen atoms is used to produce ATP as a result of these reactions. Originally, these hydrogen atoms were part of a glucose molecule. The electrons carry out the reduction of an oxygen molecule to its ions at the end of the pathway. The extra electrons on the oxygen atom attract hydrogen ions (protons) from the surrounding medium, and water is formed.
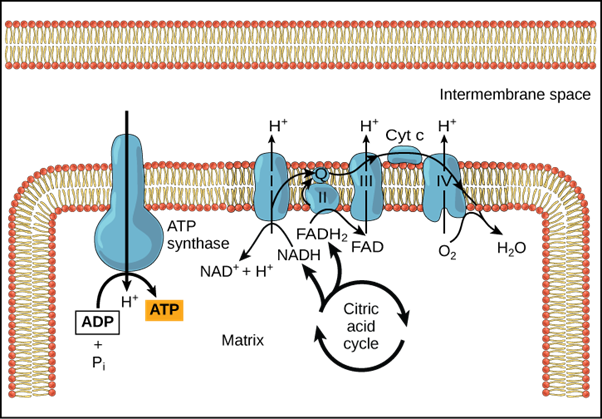
Electron Transport Chain Location
The citric acid cycle takes place in the mitochondria, which means that high-energy electrons are present there as well. As a result, in eukaryotes, the electron transport chain also occurs in the mitochondria.
The mitochondrion is a double-membraned organelle with an outer membrane and an inner membrane that is folded into cristae, a series of ridges. The matrix and the intermembrane space are the two compartments in the mitochondria.
Ions pass freely through the outer membrane. The inner membrane is impermeable to various ions and contains uncharged molecules, the electron transport chain, and ATP synthesizing enzymes, while the outer membrane contains enzymes required for the citric acid cycle.
The number of electron transport chains in mitochondria is determined by the cell's location and function. There are 10,000 sets of electron transport chains in the liver mitochondria, whereas the heart mitochondria have three times the number of electron transport chains.
The matrix contains ATP, ADP, AMP (adenosine monophosphate), NAD, NADP, and various ions such as Ca2+, Mg2+while the intermembrane space contains enzymes such as adenylate kinase.
Context and Applications
This topic is significant in the professional exams for both Bachelors and Master courses related to biology. Some of the courses are listed below:
- Bachelors in Biochemistry and Molecular Biology
- Bachelors in Zoology
- Masters in Biological Science
Want more help with your biology homework?
*Response times may vary by subject and question complexity. Median response time is 34 minutes for paid subscribers and may be longer for promotional offers.
Electron Transport Chain Homework Questions from Fellow Students
Browse our recently answered Electron Transport Chain homework questions.