results from hydrogen bonding between the backbone constituents of the overall shape of a polypeptide the polypeptide primary structure the aggregation of multiple polypeptide subunits secondary structure B pleated sheet results from interactions between side chains (R groups) of amino acids a helix tertiary structure amino acid sequence quaternary structure
results from hydrogen bonding between the backbone constituents of the overall shape of a polypeptide the polypeptide primary structure the aggregation of multiple polypeptide subunits secondary structure B pleated sheet results from interactions between side chains (R groups) of amino acids a helix tertiary structure amino acid sequence quaternary structure
Biochemistry
9th Edition
ISBN:9781319114671
Author:Lubert Stryer, Jeremy M. Berg, John L. Tymoczko, Gregory J. Gatto Jr.
Publisher:Lubert Stryer, Jeremy M. Berg, John L. Tymoczko, Gregory J. Gatto Jr.
Chapter1: Biochemistry: An Evolving Science
Section: Chapter Questions
Problem 1P
Related questions
Question
7L.6

Transcribed Image Text:Amino
acids
Val
Phe
W
Arg 35
Lys
Asp
Lys
Thr 75
Tyr
Ser
Ser
Thr
30
Primary Structure
Linear chain of amino acids
115
Thr
Amino end
80
His Val Ala Val Asn lle Ala Pro Ser Gly Arg Val Ala Asp Leu Val Lys
70
+H₂N-Gly Pro The Gly The Gly Glu Ser Lys Cys
40
25
H
45
110
20
Primary structure of transthyretin
O
Ala Ala Asp Asp The Trp Glu Pro Phe Ala Ser Gly Lys Thr Ser Glu Ser
lle Giu Val Lys Tyr lle Gly Glu Val Pho Giu Glu Glu Thr The Leu Gly
120
125
Soo
Ala Val Val Thr Asn Pro Lys Glu
105
10
50
90
15
Pro
Tyr Trp Lys Ala Leu Gly ile Ser Pro Phe His Glu His Ala Glu Val Val
100
95
Ser Tyr Pro Ser Leu Leu Ala Ala Ile The Tyr Arg Arg Pro Gly Ser Asp
.......
Val
Leu
Met
His
Carboxyl end
The primary structure of a protein is a linked series of amino
acids with a unique sequence. As an example, let's consider
transthyretin, a globular blood protein that transports vitamin
A and one of the thyroid hormones throughout the body.
Transthyretin is made up of four identical polypeptide chains,
each composed of 127 amino acids. Shown here is one of these
chains unraveled for a closer look at its primary structure. Each
of the 127 positions along the chain is occupied by one of the
20 amino acids, indicated here by its three-letter abbreviation.
edly coiled or folded in patterns that contribute to the protein's
overall shape. These coils and folds, collectively referred to as
55 Leu secondary structure, are the result of hydrogen bonds between
the repeating constituents of the polypeptide backbone (not the
amino acid side chains). Within the backbone, the oxygen atoms
have a partial negative charge, and the hydrogen atoms attached
to the nitrogens have a partial positive charge (see Figure 2.16);
therefore, hydrogen bonds can form between these atoms. Individ-
ually, these hydrogen bonds are weak, but because they are repeated
many times over a relatively long region of the polypeptide chain,
they can support a particular shape for that part of the protein.
One such secondary structure is the a helix, a delicate coil held
together by hydrogen bonding between every fourth amino acid,
shown above. Although each transthyretin polypeptide has only
one a helix region (see tertiary structure on the next page), other
globular proteins have multiple stretches of a helix separated by
nonhelical regions (see hemoglobin on the next page). Some fi-
brous proteins, such as a-keratin, the structural protein of hair,
have the a helix formation over most of their length.
Glu
Phe
Thr
Asn
Secondary Structure
Regions stabilized by hydrogen bonds between
atoms of the polypeptide backbone
Gly Most proteins have segments of their polypeptide chains repeat-
a helix
The primary structure is like the order of letters in a very long
word. If left to chance, there would be 20¹7 different ways of
making a polypeptide chain 127 amino acids long. However, the
precise primary structure of a protein is determined not by the ran-
dom linking of amino acids, but by inherited genetic information.
The primary structure in turn dictates secondary and tertiary struc-
ture, due to the chemical nature of the backbone and the side
chains (R groups) of the amino acids positioned along the chain.
3 pleated sheet
Hydrogen bond
-B strand, shown as a flat
arrow pointing toward
the carboxyl end
-Hydrogen bond
The other main type of secondary structure is the B pleated
sheet. As shown above, in this structure two or more strands of
the polypeptide chain lying side by side (called B strands) are
connected by hydrogen bonds between parts of the two parallel
polypeptide backbones. ß pleated sheets make up the core of many
globular proteins, as is the case for transthyretin (see tertiary struc-
ture on the next page), and dominate some fibrous proteins, in-
cluding the silk protein of a spider's web. The teamwork of so
many hydrogen bonds makes each spider silk fiber stronger than
a steel strand of the same weight.
Tertiary Structure
Three-dimensional shape stabilized by
interactions between side chains
Superimposed on the patterns of secondary structure is a protein's
tertiary structure, shown above in a ribbon model of the trans-
thyretin polypeptide. While secondary structure involves interac-
tions between backbone constituents, tertiary structure is the
overall shape of a polypeptide resulting from interactions between
the side chains (R groups) of the various amino acids. One type of
interaction that contributes to tertiary structure is somewhat mis-
leadingly called a hydrophobic interaction. As a polypeptide
folds into its functional shape, amino acids with hydrophobic
(nonpolar) side chains usually end up in clusters at the core of the
protein, out of contact with water. Thus, a "hydrophobic interac-
tion" is actually caused by the exclusion of nonpolar substances by
water molecules. Once nonpolar amino acid side chains are close
together, van der Waals interactions help hold them together.
Meanwhile, hydrogen bonds between polar side chains and ionic
bonds between positively and negatively charged side chains also
help stabilize tertiary structure. These are all weak interactions in
the aqueous cellular environment, but their cumulative effect helps
give the protein a unique shape.
Covalent bonds called disulfide bridges may further reinforce
the shape of a protein. Disulfide bridges form where two cysteine
monomers, which have sulfhydryl groups (-SH) on their side
chains (see Figure 4.9), are brought close together by the folding
of the protein. The sulfur of one cysteine bonds to the sulfur of the
second, and the disulfide bridge (-S-S-) rivets parts of the pro-
tein together (see yellow lines in Figure 5.18a). All of these different
kinds of interactions can contribute to the tertiary structure of at
protein, as shown here in a small part of a hypothetical protein:
OH
NHO
-Hydrogen
bond
Disulfide
bridge
H₂
Transthyretin
polypeptide
Polypeptide-
ide/
backbone
CH
CH₂ CH₂
CH₂ CH₂
-Hydrophobic
interactions and
van der Waals
interactions.
lonic bond
Quaternary Structure
Association of multiple polypeptides,
forming a functional protein
Collagen
Some proteins consist of two or more polypeptide chains aggregated
into one functional macromolecule. Quaternary structure is the
overall protein structure that results from the aggregation of these
polypeptide subunits. For example, shown above is the complete
globular transthyretin protein, made up of its four polypeptides.
Another example is collagen, shown below, which is a fibrous
protein that has three identical helical polypeptides intertwined
into a larger triple helix, giving the long fibers great strength. This
suits collagen fibers to their function as the girders of connective
tissue in skin, bone, tendons, ligaments, and other body parts.
(Collagen accounts for 40% of the protein in a human body.)
Hemoglobin, the oxygen-binding protein of red blood cells
shown below, is another example of a globular protein with
quaternary structure. It consists of four polypeptide subunits, two
of one kind (a) and two of another kind (B). Both o and ẞ subunits
consist primarily of a-helical secondary structure. Each subunit has
a nonpolypeptide component, called heme, with an iron atom that
binds oxygen.
a subunit
B subunit
Transthyretin
protein
(four identical
polypeptides)
Heme
Iron
Hemoglobin
B subunit
a subunit

Transcribed Image Text:The four levels of protein structure are primary, secondary, tertiary, and quaternary structure. Review the levels of protein structure by clicking the image below.
Drag each label into the appropriate bin depending on whether it applies to primary, secondary, tertiary, or quaternary structure.
results from hydrogen bonding
between the backbone constituents of the overall shape of a polypeptide
the polypeptide
primary structure
www
the aggregation of multiple
polypeptide subunits
secondary structure
B pleated sheet
results from interactions between side
chains (R groups) of amino acids
a helix
tertiary structure
amino acid sequence
Reset
quaternary structure
Help
Expert Solution

This question has been solved!
Explore an expertly crafted, step-by-step solution for a thorough understanding of key concepts.
This is a popular solution!
Trending now
This is a popular solution!
Step by step
Solved in 3 steps with 2 images

Recommended textbooks for you
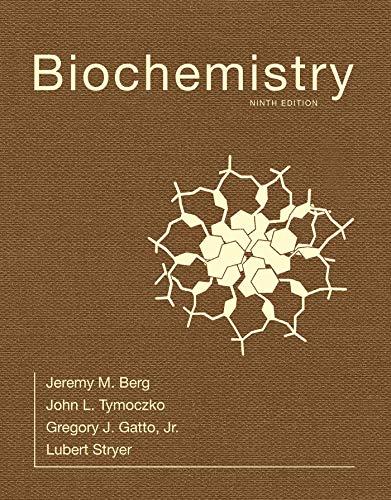
Biochemistry
Biochemistry
ISBN:
9781319114671
Author:
Lubert Stryer, Jeremy M. Berg, John L. Tymoczko, Gregory J. Gatto Jr.
Publisher:
W. H. Freeman
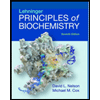
Lehninger Principles of Biochemistry
Biochemistry
ISBN:
9781464126116
Author:
David L. Nelson, Michael M. Cox
Publisher:
W. H. Freeman
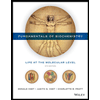
Fundamentals of Biochemistry: Life at the Molecul…
Biochemistry
ISBN:
9781118918401
Author:
Donald Voet, Judith G. Voet, Charlotte W. Pratt
Publisher:
WILEY
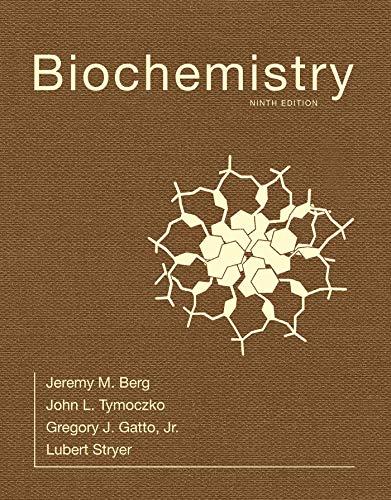
Biochemistry
Biochemistry
ISBN:
9781319114671
Author:
Lubert Stryer, Jeremy M. Berg, John L. Tymoczko, Gregory J. Gatto Jr.
Publisher:
W. H. Freeman
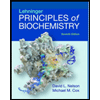
Lehninger Principles of Biochemistry
Biochemistry
ISBN:
9781464126116
Author:
David L. Nelson, Michael M. Cox
Publisher:
W. H. Freeman
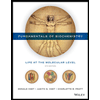
Fundamentals of Biochemistry: Life at the Molecul…
Biochemistry
ISBN:
9781118918401
Author:
Donald Voet, Judith G. Voet, Charlotte W. Pratt
Publisher:
WILEY
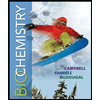
Biochemistry
Biochemistry
ISBN:
9781305961135
Author:
Mary K. Campbell, Shawn O. Farrell, Owen M. McDougal
Publisher:
Cengage Learning
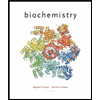
Biochemistry
Biochemistry
ISBN:
9781305577206
Author:
Reginald H. Garrett, Charles M. Grisham
Publisher:
Cengage Learning
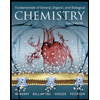
Fundamentals of General, Organic, and Biological …
Biochemistry
ISBN:
9780134015187
Author:
John E. McMurry, David S. Ballantine, Carl A. Hoeger, Virginia E. Peterson
Publisher:
PEARSON