For the NH3/H2O cycle the following relationship give the solution flow rate per unit refrigeration rate: WFS_A * X - WFS_G(X-1) = 1 where WFS_A = mass fraction of NH3 in solution form the absorber WFS_G = mass fraction of NH3 in solution from the generator X-1 =mass of solution from the generator per unit mass of refrigerant flow For large systems, a reasonable pressure drop between the evaporator and absorber is 1.5 psi Consider a large NH3/H2O plant operating per the schematic below: Known/given: i. Refrigeration load = 500 tons ii. Evaporator temperature (state point 10) = 41.1 F iii. Evaporator pressure (state point 10) = 75 psia iv. Absorber pressure (state point 11) = 73.5 psia (per rule of thumb above regarding pressure drop between the evaporator and absorber) v. Strong aqua solution temperature (state point 3) = 105 F vi. Condenser temperature (state point 8) = 100 F vii. Condenser and tower pressure (state point 7) = 211.9 psia viii. Concentration split, WFS_G - WFS_A =6% by weight (which allows for sufficient flow rate and adequate wetting of plain horizontal tubes up to 1 inch diameter in optimum arranged absorbers when using gravity feed for large systems, and it also ensures reasonable maximum liquid flows for cost effective heat exchangers and towers and a practical minimum temperature of heat source for the generator) ix. Cooing tower water temperature = 85 F Assume a 3% increase in the theoretical refrigerant flow rate to account for heat losses from the high temperature shells and heat gain through insulation for the evaporator, thus the LIFT = 1.03*500 tons = 6,180,000 BTU/hr. Next, From state point 11 & a 6% split 0.49*X – 0.43(X-1) = 1 X=9.5 X-1 = 8.5 The SA is held to w/in 3 F of equilibrium of the tower (211.9 psia & 49% aqua gives 176.9 F) to avoid flashing in the feed valve to the generator, compute the SA temperature to the tower (DEG F) i.e. 176.9 – 3 = 173.9F For the 6% split, the 43% WA exits the tower (or generator) at an equilibrium temperature of 194.9 F Refer to control volume of the tower, condenser and generator above for terminology of Ql, QC, QR, QF, QG, & QWA respectively: b. Compute the refrigerant flow rate (lb//hr) = FR = 1.03*500 TON*12000 (BTU/hr/TON)/(h10,v – h8,l) (BTU/lb) c. Ql = FR*hl,8 (BTUH) (Recall, 1 BTU/hr = 1 BTUH) d. QC = FR*(hv,7 – hl, 8) (BTUH) e. QR =0.167*FR(hv,7 – hl,8) (BTUH) (where the factor of 0.167 accounts for reflux) f. QWA = (X-1)FR*hl,1 (BTUH) g. HEAT OUT = QL + QC +QR+ QWA (BTUH) h. HEAT IN = QF = X*FR*hl,4 (BTUH) i. Generator specific heat (BTUH) = QG =HEAT IN – HEAT OUT (BTUH) j. HEAT IN TO ABSORBER= FR*hv, 10 (BTUH) + X*FR*hl,2 (BTUH) k. HEAT OUT OF ABSORBER = X*FR*hl,3 (BTUH) l. ABSOBER LOAD = QA = HEAT IN TO ABSORBER - HEAT OUT OF ABSORBER (BTUH) m. COP = LIFT / QG
For the NH3/H2O cycle the following relationship give the solution flow rate per unit refrigeration rate:
WFS_A * X - WFS_G(X-1) = 1
where
WFS_A = mass fraction of NH3 in solution form the absorber
WFS_G = mass fraction of NH3 in solution from the generator
X-1 =mass of solution from the generator per unit mass of refrigerant flow
For large systems, a reasonable pressure drop between the evaporator and absorber is 1.5 psi
Consider a large NH3/H2O plant operating per the schematic below:
Known/given:
i. Refrigeration load = 500 tons
ii. Evaporator temperature (state point 10) = 41.1 F
iii. Evaporator pressure (state point 10) = 75 psia
iv. Absorber pressure (state point 11) = 73.5 psia (per rule of thumb above regarding pressure drop between the evaporator and absorber)
v. Strong aqua solution temperature (state point 3) = 105 F
vi. Condenser temperature (state point 8) = 100 F
vii. Condenser and tower pressure (state point 7) = 211.9 psia
viii. Concentration split, WFS_G - WFS_A =6% by weight (which allows for sufficient flow rate and adequate wetting of plain horizontal tubes up to 1 inch diameter in optimum arranged absorbers when using gravity feed for large systems, and it also ensures reasonable maximum liquid flows for cost effective heat exchangers and towers and a practical minimum temperature of heat source for the generator)
ix. Cooing tower water temperature = 85 F
Assume a 3% increase in the theoretical refrigerant flow rate to account for heat losses from the high temperature shells
and heat gain through insulation for the evaporator, thus the LIFT = 1.03*500 tons = 6,180,000 BTU/hr.
Next,
From state point 11 & a 6% split
0.49*X – 0.43(X-1) = 1
X=9.5
X-1 = 8.5
The SA is held to w/in 3 F of equilibrium of the tower (211.9 psia & 49% aqua gives 176.9 F) to avoid flashing in the feed valve to the generator, compute the SA temperature to the tower (DEG F) i.e. 176.9 – 3 = 173.9F
For the 6% split, the 43% WA exits the tower (or generator) at an equilibrium temperature of 194.9 F
Refer to control volume of the tower, condenser and generator above for terminology of Ql, QC, QR, QF, QG, & QWA respectively:
b. Compute the refrigerant flow rate (lb//hr) = FR = 1.03*500 TON*12000 (BTU/hr/TON)/(h10,v – h8,l) (BTU/lb)
c. Ql = FR*hl,8 (BTUH) (Recall, 1 BTU/hr = 1 BTUH)
d. QC = FR*(hv,7 – hl, 8) (BTUH)
e. QR =0.167*FR(hv,7 – hl,8) (BTUH) (where the factor of 0.167 accounts for reflux)
f. QWA = (X-1)FR*hl,1 (BTUH)
g. HEAT OUT = QL + QC +QR+ QWA (BTUH)
h. HEAT IN = QF = X*FR*hl,4 (BTUH)
i. Generator specific heat (BTUH) = QG =HEAT IN – HEAT OUT (BTUH)
j. HEAT IN TO ABSORBER= FR*hv, 10 (BTUH) + X*FR*hl,2 (BTUH)
k. HEAT OUT OF ABSORBER = X*FR*hl,3 (BTUH)
l. ABSOBER LOAD = QA = HEAT IN TO ABSORBER - HEAT OUT OF ABSORBER (BTUH)
m. COP = LIFT / QG



Step by step
Solved in 2 steps

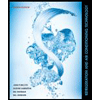
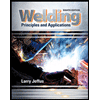
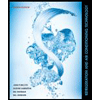
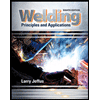