33.3 Accelerators Create Matter from Energy Before looking at all the particles we now know about, let us examine some of the machines that created them. The fundamental process in creating previously unknown particles is to accelerate known particles, such as protons or electrons, and direct a beam of them toward a target. Collisions with target nuclei provide a wealth of information, such as information obtained by Rutherford using energetic helium nuclei from natural a radiation. But if the energy of the incoming particles is large enough, new matter is sometimes created in the collision. The more energy input or AE , the more matter m can be created, since m = AE/c². Limitations are placed on what can occur by known conservation laws, such as conservation of mass-energy, momentum, and charge. Even more interesting are the unknown limitations provided by nature. Some expected reactions do occur, while others do not, and still other unexpected reactions may appear. New laws are revealed, and the vast majority of what we know about particle physics has come from accelerator laboratories. It is the particle physicist's favorite indoor sport, which is partly inspired by theory. Early Accelerators An early accelerator is a relatively simple, large-scale version of the electron gun. The Van de Graaff (named after the Dutch physicist), which you have likely seen in physics demonstrations, is a small version of the ones used for nuclear research since their invention for that purpose in 1932. For more, see Figure 33.7. These machines are electrostatic, creating potentials as great as 50 MV, and are used to accelerate a variety of nuclei for a range of experiments. Energies produced by Van de Graaffs are insufficient to produce new particles, but they have been instrumental in exploring several aspects of the nucleus. Another, equally famous, early accelerator is the cyclotron, invented in 1930 by the American physicist, E. O. Lawrence (1901–1958). For a visual representation with more detail, see Figure 33.8. Cyclotrons use fixed-frequency alternating electric fields to accelerate particles. The particles spiral outward in a magnetic field, making increasingly larger radius orbits during acceleration. This clever arrangement allows the successive addition of electric potential energy and so greater particle energies are possible than in a Van de Graaff. Lawrence was involved in many early discoveries and in the promotion of physics programs in American universities. He was awarded the 1939 Nobel Prize in Physics for the cyclotron and nuclear activations, and he has an element and two major laboratories named for him. A synchrotron is a version of a cyclotron in which the frequency of the altemating voltage and the magnetic field strength are increased as the beam particles are accelerated. Particles are made to travel the same distance in a shorter time with each cycle in fixed-radius orbits. A ring of magnets and accelerating tubes, as shown in Figure 33.9, are the major components of synchrotrons. Accelerating voltages are synchronized (i.e., occur at the same time) with the particles to accelerate them, hence the name. Magnetic field strength is increased to keep the orbital radius constant as energy increases. High-energy particles require strong magnetic fields to steer them, so superconducting magnets are commonly employed. Still limited by achievable magnetic field strengths, synchrotrons need to be very large at very high energies, since the radius of a high-energy particle's orbit is very large. Radiation caused by a magnetic field accelerating a charged particle perpendicular to its velocity is called synchrotron radiation in honor of its mportance in these machines. Synchrotron radiation has a characteristic spectrum and polarization, and can be recognized in cosmic rays, implying large-scale magnetic fields acting on energetic and charged particles in deep space. Synchrotron radiation produced by accelerators is sometimes used as a source of intense energetic electromagnetic radiation for research purposes. Figure 33.7 An artist's rendition of a Van de Grasff generator. Gap "Dees" External beam Figure 33.8 Cyclotrons use a magnetic field to cause particles to move in circular orbits. As the particles pass between the plates of the Ds, the voltage across the gap is oscillated to accelerate them twice in each orbit.
33.3 Accelerators Create Matter from Energy Before looking at all the particles we now know about, let us examine some of the machines that created them. The fundamental process in creating previously unknown particles is to accelerate known particles, such as protons or electrons, and direct a beam of them toward a target. Collisions with target nuclei provide a wealth of information, such as information obtained by Rutherford using energetic helium nuclei from natural a radiation. But if the energy of the incoming particles is large enough, new matter is sometimes created in the collision. The more energy input or AE , the more matter m can be created, since m = AE/c². Limitations are placed on what can occur by known conservation laws, such as conservation of mass-energy, momentum, and charge. Even more interesting are the unknown limitations provided by nature. Some expected reactions do occur, while others do not, and still other unexpected reactions may appear. New laws are revealed, and the vast majority of what we know about particle physics has come from accelerator laboratories. It is the particle physicist's favorite indoor sport, which is partly inspired by theory. Early Accelerators An early accelerator is a relatively simple, large-scale version of the electron gun. The Van de Graaff (named after the Dutch physicist), which you have likely seen in physics demonstrations, is a small version of the ones used for nuclear research since their invention for that purpose in 1932. For more, see Figure 33.7. These machines are electrostatic, creating potentials as great as 50 MV, and are used to accelerate a variety of nuclei for a range of experiments. Energies produced by Van de Graaffs are insufficient to produce new particles, but they have been instrumental in exploring several aspects of the nucleus. Another, equally famous, early accelerator is the cyclotron, invented in 1930 by the American physicist, E. O. Lawrence (1901–1958). For a visual representation with more detail, see Figure 33.8. Cyclotrons use fixed-frequency alternating electric fields to accelerate particles. The particles spiral outward in a magnetic field, making increasingly larger radius orbits during acceleration. This clever arrangement allows the successive addition of electric potential energy and so greater particle energies are possible than in a Van de Graaff. Lawrence was involved in many early discoveries and in the promotion of physics programs in American universities. He was awarded the 1939 Nobel Prize in Physics for the cyclotron and nuclear activations, and he has an element and two major laboratories named for him. A synchrotron is a version of a cyclotron in which the frequency of the altemating voltage and the magnetic field strength are increased as the beam particles are accelerated. Particles are made to travel the same distance in a shorter time with each cycle in fixed-radius orbits. A ring of magnets and accelerating tubes, as shown in Figure 33.9, are the major components of synchrotrons. Accelerating voltages are synchronized (i.e., occur at the same time) with the particles to accelerate them, hence the name. Magnetic field strength is increased to keep the orbital radius constant as energy increases. High-energy particles require strong magnetic fields to steer them, so superconducting magnets are commonly employed. Still limited by achievable magnetic field strengths, synchrotrons need to be very large at very high energies, since the radius of a high-energy particle's orbit is very large. Radiation caused by a magnetic field accelerating a charged particle perpendicular to its velocity is called synchrotron radiation in honor of its mportance in these machines. Synchrotron radiation has a characteristic spectrum and polarization, and can be recognized in cosmic rays, implying large-scale magnetic fields acting on energetic and charged particles in deep space. Synchrotron radiation produced by accelerators is sometimes used as a source of intense energetic electromagnetic radiation for research purposes. Figure 33.7 An artist's rendition of a Van de Grasff generator. Gap "Dees" External beam Figure 33.8 Cyclotrons use a magnetic field to cause particles to move in circular orbits. As the particles pass between the plates of the Ds, the voltage across the gap is oscillated to accelerate them twice in each orbit.
College Physics
11th Edition
ISBN:9781305952300
Author:Raymond A. Serway, Chris Vuille
Publisher:Raymond A. Serway, Chris Vuille
Chapter1: Units, Trigonometry. And Vectors
Section: Chapter Questions
Problem 1CQ: Estimate the order of magnitude of the length, in meters, of each of the following; (a) a mouse, (b)...
Related questions
Question
Accelerators Create Matter from Energy
• State the principle of a cyclotron.
• Explain the principle of a synchrotron.
• Describe the voltage needed by an accelerator between accelerating tubes.
• State Fermilab’s accelerator principle

Transcribed Image Text:33.3 Accelerators Create Matter from Energy
Before looking at all the particles we now know about, let us examine some of the machines that created them. The fundamental
process in creating previously unknown particles is to accelerate known particles, such as protons or electrons, and direct a
beam of them toward a target. Collisions with target nuclei provide a wealth of information, such as information obtained by
Rutherford using energetic helium nuclei from natural a radiation. But if the energy of the incoming particles is large enough,
new matter is sometimes created in the collision. The more energy input or AE , the more matter m can be created, since
m = AE/c². Limitations are placed on what can occur by known conservation laws, such as conservation of mass-energy,
momentum, and charge. Even more interesting are the unknown limitations provided by nature. Some expected reactions do
occur, while others do not, and still other unexpected reactions may appear. New laws are revealed, and the vast majority of
what we know about particle physics has come from accelerator laboratories. It is the particle physicist's favorite indoor sport,
which is partly inspired by theory.
Early Accelerators
An early accelerator is a relatively simple, large-scale version of the electron gun. The Van de Graaff (named after the Dutch
physicist), which you have likely seen in physics demonstrations, is a small version of the ones used for nuclear research since
their invention for that purpose in 1932. For more, see Figure 33.7. These machines are electrostatic, creating potentials as
great as 50 MV, and are used to accelerate a variety of nuclei for a range of experiments. Energies produced by Van de Graaffs
are insufficient to produce new particles, but they have been instrumental in exploring several aspects of the nucleus. Another,
equally famous, early accelerator is the cyclotron, invented in 1930 by the American physicist, E. O. Lawrence (1901–1958).
For a visual representation with more detail, see Figure 33.8. Cyclotrons use fixed-frequency alternating electric fields to
accelerate particles. The particles spiral outward in a magnetic field, making increasingly larger radius orbits during acceleration.
This clever arrangement allows the successive addition of electric potential energy and so greater particle energies are possible
than in a Van de Graaff. Lawrence was involved in many early discoveries and in the promotion of physics programs in American

Transcribed Image Text:universities. He was awarded the 1939 Nobel Prize in Physics for the cyclotron and nuclear activations, and he has an element
and two major laboratories named for him.
A synchrotron is a version of a cyclotron in which the frequency of the altemating voltage and the magnetic field strength are
increased as the beam particles are accelerated. Particles are made to travel the same distance in a shorter time with each cycle
in fixed-radius orbits. A ring of magnets and accelerating tubes, as shown in Figure 33.9, are the major components of
synchrotrons. Accelerating voltages are synchronized (i.e., occur at the same time) with the particles to accelerate them, hence
the name. Magnetic field strength is increased to keep the orbital radius constant as energy increases. High-energy particles
require strong magnetic fields to steer them, so superconducting magnets are commonly employed. Still limited by achievable
magnetic field strengths, synchrotrons need to be very large at very high energies, since the radius of a high-energy particle's
orbit is very large. Radiation caused by a magnetic field accelerating a charged particle perpendicular to its velocity is called
synchrotron radiation in honor of its mportance in these machines. Synchrotron radiation has a characteristic spectrum and
polarization, and can be recognized in cosmic rays, implying large-scale magnetic fields acting on energetic and charged
particles in deep space. Synchrotron radiation produced by accelerators is sometimes used as a source of intense energetic
electromagnetic radiation for research purposes.
Figure 33.7 An artist's rendition of a Van de Grasff generator.
Gap
"Dees"
External beam
Figure 33.8 Cyclotrons use a magnetic field to cause particles to move in circular orbits. As the particles pass between the plates of the Ds, the voltage
across the gap is oscillated to accelerate them twice in each orbit.
Expert Solution

This question has been solved!
Explore an expertly crafted, step-by-step solution for a thorough understanding of key concepts.
This is a popular solution!
Trending now
This is a popular solution!
Step by step
Solved in 2 steps with 1 images

Knowledge Booster
Learn more about
Need a deep-dive on the concept behind this application? Look no further. Learn more about this topic, physics and related others by exploring similar questions and additional content below.Recommended textbooks for you
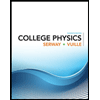
College Physics
Physics
ISBN:
9781305952300
Author:
Raymond A. Serway, Chris Vuille
Publisher:
Cengage Learning
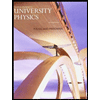
University Physics (14th Edition)
Physics
ISBN:
9780133969290
Author:
Hugh D. Young, Roger A. Freedman
Publisher:
PEARSON
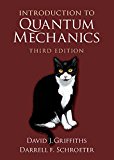
Introduction To Quantum Mechanics
Physics
ISBN:
9781107189638
Author:
Griffiths, David J., Schroeter, Darrell F.
Publisher:
Cambridge University Press
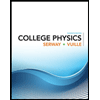
College Physics
Physics
ISBN:
9781305952300
Author:
Raymond A. Serway, Chris Vuille
Publisher:
Cengage Learning
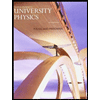
University Physics (14th Edition)
Physics
ISBN:
9780133969290
Author:
Hugh D. Young, Roger A. Freedman
Publisher:
PEARSON
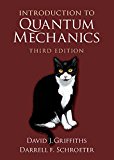
Introduction To Quantum Mechanics
Physics
ISBN:
9781107189638
Author:
Griffiths, David J., Schroeter, Darrell F.
Publisher:
Cambridge University Press
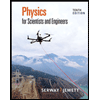
Physics for Scientists and Engineers
Physics
ISBN:
9781337553278
Author:
Raymond A. Serway, John W. Jewett
Publisher:
Cengage Learning
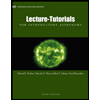
Lecture- Tutorials for Introductory Astronomy
Physics
ISBN:
9780321820464
Author:
Edward E. Prather, Tim P. Slater, Jeff P. Adams, Gina Brissenden
Publisher:
Addison-Wesley
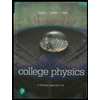
College Physics: A Strategic Approach (4th Editio…
Physics
ISBN:
9780134609034
Author:
Randall D. Knight (Professor Emeritus), Brian Jones, Stuart Field
Publisher:
PEARSON