17.4 Doppler Effect and Sonic Booms The characteristic sound of a motorcycle buzzing by is an example of the Doppler effect. The high-pitch scream shifts dramatically to a lower-pitch roar as the motorcycle passes by a stationary observer. The closer the motorcycle brushes by, the more abrupt the shift. The faster the motorcycle moves, the greater the shift. We also hear this characteristic shift in frequency for passing race cars, airplanes, and trains. It is so familiar that it is used to imply motion and children often mimic it in play. The Doppler effect is an alteration in the observed frequency of a sound due to motion of either the source or the observer. Although less familiar, this effect is easily noticed for a stationary source and moving observer. For example, if you ride a train past a stationary warning bell, you will hear the bell's frequency shift from high to low as you pass by. The actual change in frequency due to relative motion of source and observer is called a Doppler shift. The Doppler effect and Doppler shift are named for the Austrian physicist and mathematician Christian Johann Doppler (1803–1853), who did experiments with both moving sources and moving observers. Doppler, for example, had musicians play on a moving open train car and also play standing next to the train tracks as a train passed by. Their music was observed both on and off the train, and changes in frequency were measured. What causes the Doppler shift? Figure 17.14, Figure 17.15, and Figure 17.16 compare sound waves emitted by stationary and moving sources in a stationary air mass. Each disturbance spreads out spherically from the point where the sound was emitted. If the source is stationary, then all of the spheres representing the air compressions in the sound wave centered on the same point, and the stationary observers on either side see the same wavelength and frequency as emitted by the source, as in Figure 17.14. If the source is moving, as in Figure 17.15, then the situation is different. Each compression of the air moves out in a sphere from the point where it was emitted, but the point of emission moves. This moving emission point causes the air compressions to be closer together on one side and farther apart on the other. Thus, the wavelength is shorter in the direction the source is moving (on the right in Figure 17.15), and longer in the opposite direction (on the left in Figure 17.15). Finally. if the observers move, as in Figure 17.16, the frequency at which they receive the compressions changes. The observer moving toward the source receives them at a higher frequency, and the person moving away from the source receives them at a lower frequency. х Figure 17.14 Sounds emitted by a source spread out in spherical waves. Because the source, observers, and air are stationary, the wavelength and frequency are the same in all directions and to all observers. 1234 5 Figure 17.15 Sounds emitted by a source moving to the right spread out from the points at which they were emitted. The wavelength is reduced and, consequently, the frequency is increased in the direction of motion, so that the observer on the right hears a higher-pitch sound. The opposite is true for the observer on the left, where the wavelength is increased and the frequency is reduced. х Figure 17.16 The same effect is produced when the observers move relative to the source. Motion toward the source increases frequency as the observer on the right passes through more wave crests than she would if stationary. Motion away from the source decreases frequency as the observer on the left passes through fewer wave crests than he would if stationary.
17.4 Doppler Effect and Sonic Booms The characteristic sound of a motorcycle buzzing by is an example of the Doppler effect. The high-pitch scream shifts dramatically to a lower-pitch roar as the motorcycle passes by a stationary observer. The closer the motorcycle brushes by, the more abrupt the shift. The faster the motorcycle moves, the greater the shift. We also hear this characteristic shift in frequency for passing race cars, airplanes, and trains. It is so familiar that it is used to imply motion and children often mimic it in play. The Doppler effect is an alteration in the observed frequency of a sound due to motion of either the source or the observer. Although less familiar, this effect is easily noticed for a stationary source and moving observer. For example, if you ride a train past a stationary warning bell, you will hear the bell's frequency shift from high to low as you pass by. The actual change in frequency due to relative motion of source and observer is called a Doppler shift. The Doppler effect and Doppler shift are named for the Austrian physicist and mathematician Christian Johann Doppler (1803–1853), who did experiments with both moving sources and moving observers. Doppler, for example, had musicians play on a moving open train car and also play standing next to the train tracks as a train passed by. Their music was observed both on and off the train, and changes in frequency were measured. What causes the Doppler shift? Figure 17.14, Figure 17.15, and Figure 17.16 compare sound waves emitted by stationary and moving sources in a stationary air mass. Each disturbance spreads out spherically from the point where the sound was emitted. If the source is stationary, then all of the spheres representing the air compressions in the sound wave centered on the same point, and the stationary observers on either side see the same wavelength and frequency as emitted by the source, as in Figure 17.14. If the source is moving, as in Figure 17.15, then the situation is different. Each compression of the air moves out in a sphere from the point where it was emitted, but the point of emission moves. This moving emission point causes the air compressions to be closer together on one side and farther apart on the other. Thus, the wavelength is shorter in the direction the source is moving (on the right in Figure 17.15), and longer in the opposite direction (on the left in Figure 17.15). Finally. if the observers move, as in Figure 17.16, the frequency at which they receive the compressions changes. The observer moving toward the source receives them at a higher frequency, and the person moving away from the source receives them at a lower frequency. х Figure 17.14 Sounds emitted by a source spread out in spherical waves. Because the source, observers, and air are stationary, the wavelength and frequency are the same in all directions and to all observers. 1234 5 Figure 17.15 Sounds emitted by a source moving to the right spread out from the points at which they were emitted. The wavelength is reduced and, consequently, the frequency is increased in the direction of motion, so that the observer on the right hears a higher-pitch sound. The opposite is true for the observer on the left, where the wavelength is increased and the frequency is reduced. х Figure 17.16 The same effect is produced when the observers move relative to the source. Motion toward the source increases frequency as the observer on the right passes through more wave crests than she would if stationary. Motion away from the source decreases frequency as the observer on the left passes through fewer wave crests than he would if stationary.
College Physics
11th Edition
ISBN:9781305952300
Author:Raymond A. Serway, Chris Vuille
Publisher:Raymond A. Serway, Chris Vuille
Chapter1: Units, Trigonometry. And Vectors
Section: Chapter Questions
Problem 1CQ: Estimate the order of magnitude of the length, in meters, of each of the following; (a) a mouse, (b)...
Related questions
Question
Doppler Effect and Sonic Booms
• Define Doppler effect, Doppler shift, and sonic boom.
• Calculate the frequency of a sound heard by someone observing Doppler shift.
• Describe the sounds produced by objects moving faster than the speed of sound.

Transcribed Image Text:17.4 Doppler Effect and Sonic Booms
The characteristic sound of a motorcycle buzzing by is an example of the Doppler effect. The high-pitch scream shifts
dramatically to a lower-pitch roar as the motorcycle passes by a stationary observer. The closer the motorcycle brushes by, the
more abrupt the shift. The faster the motorcycle moves, the greater the shift. We also hear this characteristic shift in frequency
for passing race cars, airplanes, and trains. It is so familiar that it is used to imply motion and children often mimic it in play.
The Doppler effect is an alteration in the observed frequency of a sound due to motion of either the source or the observer.
Although less familiar, this effect is easily noticed for a stationary source and moving observer. For example, if you ride a train
past a stationary warning bell, you will hear the bell's frequency shift from high to low as you pass by. The actual change in
frequency due to relative motion of source and observer is called a Doppler shift. The Doppler effect and Doppler shift are
named for the Austrian physicist and mathematician Christian Johann Doppler (1803–1853), who did experiments with both
moving sources and moving observers. Doppler, for example, had musicians play on a moving open train car and also play
standing next to the train tracks as a train passed by. Their music was observed both on and off the train, and changes in
frequency were measured.
What causes the Doppler shift? Figure 17.14, Figure 17.15, and Figure 17.16 compare sound waves emitted by stationary and
moving sources in a stationary air mass. Each disturbance spreads out spherically from the point where the sound was emitted.
If the source is stationary, then all of the spheres representing the air compressions in the sound wave centered on the same

Transcribed Image Text:point, and the stationary observers on either side see the same wavelength and frequency as emitted by the source, as in Figure
17.14. If the source is moving, as in Figure 17.15, then the situation is different. Each compression of the air moves out in a
sphere from the point where it was emitted, but the point of emission moves. This moving emission point causes the air
compressions to be closer together on one side and farther apart on the other. Thus, the wavelength is shorter in the direction
the source is moving (on the right in Figure 17.15), and longer in the opposite direction (on the left in Figure 17.15). Finally. if the
observers move, as in Figure 17.16, the frequency at which they receive the compressions changes. The observer moving
toward the source receives them at a higher frequency, and the person moving away from the source receives them at a lower
frequency.
х
Figure 17.14 Sounds emitted by a source spread out in spherical waves. Because the source, observers, and air are stationary, the wavelength and
frequency are the same in all directions and to all observers.
1234 5
Figure 17.15 Sounds emitted by a source moving to the right spread out from the points at which they were emitted. The wavelength is reduced and,
consequently, the frequency is increased in the direction of motion, so that the observer on the right hears a higher-pitch sound. The opposite is true for
the observer on the left, where the wavelength is increased and the frequency is reduced.
х
Figure 17.16 The same effect is produced when the observers move relative to the source. Motion toward the source increases frequency as the
observer on the right passes through more wave crests than she would if stationary. Motion away from the source decreases frequency as the observer
on the left passes through fewer wave crests than he would if stationary.
Expert Solution

This question has been solved!
Explore an expertly crafted, step-by-step solution for a thorough understanding of key concepts.
This is a popular solution!
Trending now
This is a popular solution!
Step by step
Solved in 4 steps with 4 images

Knowledge Booster
Learn more about
Need a deep-dive on the concept behind this application? Look no further. Learn more about this topic, physics and related others by exploring similar questions and additional content below.Recommended textbooks for you
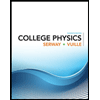
College Physics
Physics
ISBN:
9781305952300
Author:
Raymond A. Serway, Chris Vuille
Publisher:
Cengage Learning
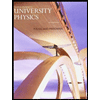
University Physics (14th Edition)
Physics
ISBN:
9780133969290
Author:
Hugh D. Young, Roger A. Freedman
Publisher:
PEARSON
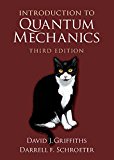
Introduction To Quantum Mechanics
Physics
ISBN:
9781107189638
Author:
Griffiths, David J., Schroeter, Darrell F.
Publisher:
Cambridge University Press
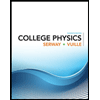
College Physics
Physics
ISBN:
9781305952300
Author:
Raymond A. Serway, Chris Vuille
Publisher:
Cengage Learning
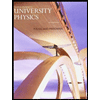
University Physics (14th Edition)
Physics
ISBN:
9780133969290
Author:
Hugh D. Young, Roger A. Freedman
Publisher:
PEARSON
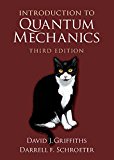
Introduction To Quantum Mechanics
Physics
ISBN:
9781107189638
Author:
Griffiths, David J., Schroeter, Darrell F.
Publisher:
Cambridge University Press
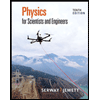
Physics for Scientists and Engineers
Physics
ISBN:
9781337553278
Author:
Raymond A. Serway, John W. Jewett
Publisher:
Cengage Learning
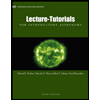
Lecture- Tutorials for Introductory Astronomy
Physics
ISBN:
9780321820464
Author:
Edward E. Prather, Tim P. Slater, Jeff P. Adams, Gina Brissenden
Publisher:
Addison-Wesley
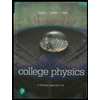
College Physics: A Strategic Approach (4th Editio…
Physics
ISBN:
9780134609034
Author:
Randall D. Knight (Professor Emeritus), Brian Jones, Stuart Field
Publisher:
PEARSON