Lab 12 Human Genetics
docx
keyboard_arrow_up
School
South Texas College *
*We aren’t endorsed by this school
Course
1408
Subject
Biology
Date
Dec 6, 2023
Type
docx
Pages
29
Uploaded by UltraProton9047
Note: All your answers to questions must be in Red or other color (not
including blue) for easier grading. Points will be deducted if you do not
distinguish your answers.
Lab 12. Patterns of Inheritance
Objectives:
Determine
the genotype by observation of individuals with given
traits and their relatives.
Determine
inheritance involving autosomal dominant, autosomal
recessive and x-linked recessive alleles.
Determine
inheritance involving multiple allele inheritance and use
blood type to help determine paternity.
Describe
a normal human karyotype and discuss the various
abnormalities that can be detected using this technique
Using a karyotype
analyze
a patient’s chromosomes to determine if
inheritance is normal or a chromosomal anomaly is present.
Analyze
a pedigree to determine the pattern of inheritance for an
allele – autosomal dominant, autosomal recessive or x-linked recessive.
Create
a pedigree to determine the probability of inheritance of a
particular phenotype when given generational information only.
Vocabulary:
Genotype
Phenotype
Autosomal dominant
Autosomal recessive
Heterozygous
Homozygous
Probability
X-linked recessive
X-linked dominant
Multiple alleles
Codominance
Karyotype
Pedigree
Introduction:
Just like in pea plants, Mendel’s laws of inheritance also apply to
humans. Humans inherit 46 chromosomes that occur in 23 pairs, 22 pairs of
autosomes
and one pair of
sex chromosomes
(either XX or XY). This
means that all autosomal genes exist in two forms, called alleles. These
alleles may be genetically identical, called
homozygous
(AA or aa) or they
may be genetically different (Aa or aA). The fact that one allele may be
dominant over the other determines what phenotype is exhibited.
Dominant
alleles are represented by capital letters (A) and
recessive
are represented
by lower case (a). Thus, you can have
homozygous dominant
(AA) or
homozygous recessive
(aa) and of course
heterozygous
(Aa).
When a gene follows Mendel’s laws of inheritance (MOST genes do
not), it is easy to predict the ratios of potential offspring using Punnett
squares, or for example to determine the genotypes of parents from the
genotype or phenotype of the offspring. In this section of the lab, we will
practice determining the genotype given information about the gene and
inheritance of it.
Part 1: Determining Genotype
Table 1
shows several autosomal human traits and indicates which is
dominant and which is recessive. Fill out Table 1 and answer the following questions.
(If you are not sure what the trait looks like, refer to
Figure 1-5
, or you can do a
quick internet search for an image of that trait.)
Figure 1:
Left – Widow's peak (dominant) versus Right– straight hairline (recessive).
Figure 2:
Left – No Hitchhiker’s thumb (dominant) versus Right– Hitchhiker’s thumb (recessive).
Figure 3:
Right
– attached earlobes (recessive) versus Left– unattached earlobes (dominant).
Figure 4:
Left– freckles (dominant) versus Right– no freckles (recessive).
Figure 5:
Left– dimples(dominant) versus Right– no dimples (recessive).
Your preview ends here
Eager to read complete document? Join bartleby learn and gain access to the full version
- Access to all documents
- Unlimited textbook solutions
- 24/7 expert homework help
Trait
(Capital letter – dominant allele)
(Lowercase letter– recessive allele)
Possible
Genotype
s
Your
Phenoty
pe
Your
Genotyp
e
Widow’s peak – W
Straight
hairline – w
WW, Ww,
ww
Widow’s
peak
Ww
Earlobes unattached – U
Earlobes attached – u
UU, Uu, uu
Earlobes
unattach
ed
Uu
Skin pigmentation:
Freckles – F
No freckles – f
FF. Ff,
ff
No
freckles
ff
Hair on back of hand – H
NO hair on back of hand – h
HH, Hh, hh
No hair
on back
of hand
hh
Dimples – D
No Dimples – d
DD, Dd,dd
No
dimples
dd
Polydactyly (more than 5 fingers)
– P
Five fingers – p
PP ,Pp, pp
Five
fingers
pp
Thumb hyperextension –
“hitchhiker’s thumb”
Last segment cannot be bent
backward – T
Last segment can be bent
backward - t
TT, Tt, tt
Last
segment
can’t
bent
backwar
d
Tt
Questions:
1.
What is the homozygous recessive genotype for dimples? What is the
phenotype?
The homozygous recessive is dd. The phenotype is no
dimples.
2.
Does an individual with Pp exhibit the symptoms of polydactyly (more
than 5 fingers)? Why or why not?
Yes, any person with polydactyly has the
genotype of Pp, where P represents the allele that causes polydactyly and
p presents the normal allele of this gene.
3.
What is the genotype for an individual who is heterozygous for
hitchhiker’s thumb? Will they exhibit the phenotype for hitchhiker’s
thumb?
The genotype for someone who is heterozygous for hitchhiker’s thumb is
Tt. They will not exhibit the hitchhiker’s thumb phenotype instead they
will have a straight thumb.
4.
Two people who are heterozygous for earlobes unattached have a child.
List the genotypes possible for their children regarding earlobe
attachment. Show your work.
UU (for earlobes unattached)
Uu (for earlobes unattached)
Uu (for earlobes unattached)
uu (for earlobes attached)
5.
Ryann does not have freckles, but both their parent’s do. Deduce the genotype
of their parents. What is Ryann’s genotype?
The parents genotype are FF and Ff. Ryann’s genotype is ff.
6.
Godric is heterozygous for widow’s peak and has no hair on the back of his hand.
His partner has a straight hairline and is heterozygous for hair on the back of
their hand.
a.
What is Godric’s genotype?
Ww , hh
(heterozygous widow’s peak; no
hair back of hand)
b.
What is his partner’s genotype?
ww , Hh (straight hairline,
heterozygous-hair back of hand)
c.
What possible genotypes will their children have for hair lines?
WW,
Ww, ww
d.
What possible genotypes will their children have for hair on the back of
the hand?
Hh, hh
Part 2: A Taste of Genetics – Life Example with Taste
Every organism on Earth has a different way to perceive the world due
to their individual life experiences as well as their genetic make-up. Humans
are no different; every individual has their own experiences that shapes their
world perception but so too does their DNA. You may be surprised to learn
that 99.9% of the human genome is identical from one individual to the next,
and it is the 0.1% difference that makes each individual unique.
Some of these differences can affect our sensory systems and how we
perceive the natural world. For example, over time we have learned which
things taste good and are good for us while simultaneously learning which
things taste bad or are bad for us. Specifically, bitter compounds are closely
associated to toxic substances in nature. The way we know things taste
bitter, or any other flavor for that matter, is because we have special
chemical receptors in our mouth and nose that bind molecules in our food
and send signals to the brain telling it what the food tastes like.
Figure 6:
A chemical binding a membrane receptor
One type of bitter receptor in our mouth senses the presence of a
chemical called phenylthiocabamide, or PTC. PTC is a non-toxic chemical, but
it very closely resembles toxic compounds often found in food. The unique
thing about PTC is that not everyone can taste it! We first learned this in the
1920s when Arthur L. Fox and C. R. Noller were working with PTC powder and
Noller complained about the extremely bitter taste while Fox tasted nothing
at all. This led to experimentation where scientists ultimately discovered the
ability to taste PTC was hereditary; it was in our DNA!
The ability to taste PTC comes from the gene
TAS2R38
which encodes
one of the chemical receptors in our mouth that binds to PTC. By comparing
PTC tasters to non-tasters, scientists have found three single nucleotide
polymorphisms (SNPs)
that differentiate the taster allele (T) from the non-
taste allele (t). A SNP is a genetic mutation where one nucleotide in DNA is
different from one individual to the next. The word mutation sounds scary,
but a mutation is not always bad; there are nearly 10 million SNPs in humans
Your preview ends here
Eager to read complete document? Join bartleby learn and gain access to the full version
- Access to all documents
- Unlimited textbook solutions
- 24/7 expert homework help
which means SNPs are common. The three SNPs (see table 1) found in
the
TAS2R38
gene leads to changes in the amino acid sequence which can
potentially change the proteins’ function.
Table 1: SNPs Present in Tasters vs Non-Tasters for PTC
Nucleoti
de
Position
(bp)
Nucleotide
Change
Codon Change
Amino Acid Change
Phenoty
pe
Non-
Taster
Taster
Non-
Taster
Taster
Non-
taster
Taster
145
G
C
G
CA
C
CA
Alanine
Proline
785
T
B
G
T
T
G
C
T
Valine
Alanine
886
A
G
A
TC
G
TC
Isoleucin
e
Valine
Individuals who are tasters can be TT (homozygous dominant) or Tt
(heterozygous). Individuals who are non-tasters will always be tt
(homozygous recessive). To understand how the genes are inherited,
examine table 2 below where the potential offspring of two heterozygous
parents are analyzed. There is a 75% chance of having children that are
tasters for PTC and a 25% chance of having children that are non-tasters.
Table 2. Sample Inheritance Pattern for PTC Tasting
Parent
Alleles
T
T
T
TT
(homozygous
taster)
Tt (heterozygous
taster)
t
Tt
(heterozygous
taster)
Tt (homozygous
non-taster)
In this section of the lab, you will use a set of data that was collected
from a previous class. This data has not yet been analyzed, therefore you will
have to sort through it and determine what conclusions, if any, can be made.
To collect this data, 35 participants were asked to place a strip of PTC
paper (paper coated with
phenylthiocabamide) on their tongue. As soon as they
detected a taste, they were instructed to remove the paper and report what the
taste was, or if they never detected any taste. Those who reported that it was
extremely bitter, “to the point of almost not being able to take it” as several
commented, were also recorded. It is normal that some individuals who are tasters
are also much more sensitive to PTC and thus sense the bitterness much more
strongly than normal tasters. We call these individuals “Super tasters”.
Data:
Table 3: Results from one class (35 different individuals) who
conducted a PTC taste test.
Participa
nt
No
Taste
Tasted
Bitter
Extremely Bitter (Super
Taster)
1
x
2
x
3
x
4
x
x
5
x
6
x
x
7
x
8
x
9
x
x
10
x
11
x
12
x
x
13
x
14
x
15
x
16
x
x
17
x
18
x
19
x
20
x
21
x
22
x
23
x
24
x
x
25
x
26
x
27
x
28
x
29
x
30
x
x
31
x
32
x
33
x
x
34
x
35
x
Your preview ends here
Eager to read complete document? Join bartleby learn and gain access to the full version
- Access to all documents
- Unlimited textbook solutions
- 24/7 expert homework help
Questions:
7.
What is the total number of non-tasters in the class?
9
8.
What is the
percent
of the class that are non-tasters of PTC?
9/35 x
100=25.7%
9.
What is the genotype on non-tasters?
tt
10.
What is the total number of tasters in the class?
26
11.
What is the
percent
of the class that are tasters of PTC?
74.2%
12.
What is the genotype(s) of tasters?
The genotype of tasters is TT or Tt
13.
Given that some tasters are super-tasters, what is the
percentage of
the class
that are super tasters?
22.9%
14.
Individuals that are known as “Super tasters” are thought to have more of the
protein which binds to and detects PTC on their tongues compared to tasters.
Given this information, what genotype do you think that Supertasters are and
why?
Supertasters genotype would be TT. They have much more sensitivity due
to the gene
TAS2R38
which encodes the chemical receptors in the mouth that
binds to PTC.
15.
If two parents, one heterozygote and one homozygous dominant for
PTC tasting were to have children, what are all the possible genotypes and
phenotypes?
Parents genotype:
Tt
(heterozygous) and TT
(homozygous dominant)
Genotypes:
(homozygous) 50% chance of a child being TT
(heterozygous) 50% chance of a child being Tt
(Tasters) 100% chance all children being able to taste PTC
Phenotypes:
(Taster) this results from both the TT and Tt genotypes since the
presence of at least one
dominant allele T confers the ability to taste PTC.
Part 3: Determining Inheritance
Figure 7:
Two Punnett squares showing possible sperm on the vertical edge and possible eggs on the
horizontal edge. All boxes are filled in according to the alleles contributed by the sperm and egg.
This Photo
by Unknown Author is licensed under
CC BY
3.0
Recall that using a Punnett square allows you to determine all the possible
genotype combinations that offspring could inherit from two parents. In a
Punnett square all possible types of sperm are lined up on one side of the
square and all types of eggs are lined up on the other. Then the squares are
filled in according to what each sperm and egg would give to the resulting
zygote. By analyzing all the resulting genotypes, it is possible to calculate
the
probability
of each genotype occurring.
Figure 7 shows two Punnett squares with all possible sperm displayed
on the vertical edge and all possible eggs on the horizontal edge. All boxes
have been filled in according to the alleles contributed by the sperm and egg
for that box. We can now calculate the probabilities of each genotype and
phenotype. All probabilities are out of 4, as this is a 4 square box.
For the left Punnett square, the single box filled
with genotype bb is
highlighted in red. As this is the only
bb present it has a probability of 1 in 4,
¼, or 25%. This is both the genotypic and phenotypic ratio.
For the right Punnett square there are
three boxes highlighted in red,
indicating the
dominant phenotypes.
Because there are three of the four
boxes the phenotypic ratio is 3 of 4, ¾, or 75%. This is not the genotypic
ratio however as there are two genotypes included in these three selected
squares.
The genotypic ratio for that Punnett square would be a
1:2:1 ratio.
Questions:
16.
Fill in the Punnett square:
a
a
A
Aa
Aa
a
aa
aa
17.
Refer to question 16 above.
a.
What is the probability of having offspring with a genotype of aa?
2/4 or 50%
b.
What is the probability of having offspring with a genotype of
AA?
0
c.
What is the probability of dominant phenotype?
¾ or 75%
18.
In guinea pigs, the
allele for short hair is
dominant to long hair
.
d.
What genotype would a heterozygous short
haired guinea pig have?
Ss
e.
What genotype would a homozygous short
haired guinea pig have?
SS or Ss
f.
What genotype would a long-haired guinea pig
have?
ss
g.
Show the cross for two heterozygous guinea pigs.
_S
_
_s
_
_S
_
SS
Ss
_s
_
Ss
ss
What percentage of the offspring will have short hair?
75%
long
hair?
25%
19.
In guinea pigs curly hair is dominant to straight. Show the cross
between two homozygous curly haired guinea pigs.
_C
_
_C
_
_C
_
CC
CC
_C
_
CC
CC
What percentage of the offspring will have curly hair?
100%
Your preview ends here
Eager to read complete document? Join bartleby learn and gain access to the full version
- Access to all documents
- Unlimited textbook solutions
- 24/7 expert homework help
20.
Punnett squares can also be used to predict genotypes of the parents.
A guinea pig with short hair is crossed to one that has long hair. Over a
period of years, a scientist keeps records of the offspring and finds that 44
of them have curly hair and 46 of them have straight hair. Based on this
data, what are the genotypes of the parents:
_CC_
x
_cc_
Show the Punnett square of this cross to explain your
reasoning.
Genetic counselors are trained to detect inheritance
patterns of genetic diseases based on information they
obtain from the family. Imagine that you are a genetic
counselor, and you must solve the following cases based on the information
provided. Use the following steps to solve each problem:
Create a legend that indicates the gene pairs (alleles) involved. Use a
capital letter to denote the dominant allele and lowercase letter to
denote the recessive allele.
Example: D= dimples d= no dimples
C = Curly hair (dominant)
c = Straight hair (recessive)
Write the genotype and phenotype of the parents. Example: DD →
dimples
CC or Cc
curly hair
cc
straight hair
Use a Punnett Square to cross the potential gametes of the parents.
Determine the probability based on Punnett Square.
25% probability offspring having CC
50% probability offspring having Cc
25% probability offspring having cc
Autosomal Disorders:
21.
Autosomal Recessive Inheritance (trait only expressed if
homozygous recessive)
An albino man (nn) marries a normally pigmented woman (N_) who has an
albino mother (nn). What is the chance that their children will be albino?
_C
_
_c
_
c
_
Cc
cc
_c
_
CC
cc
The chance that their children will be albino is 50%. The children will be
carriers of the albino gene (Nn).
22.
Autosomal Dominant Inheritance (trait expressed as long as
one dominant allele is present)
A daughter wants to know what the chances are that she will develop
Huntington’s disease, a degenerative disorder of the nervous system that
appears during the ages of 30s to 40s. Her mother has Huntington’s while
her father does not have the disease. Try to determine the possibilities
from the information you have at hand. What further information do you
need in order to more accurately determine the probability?
The
daughter’s genotype could be one of the following: Hh (inherited the
mutated gene from her mother) or hh (inherited the normal gene from
both parents).
By inheriting the mutated gene (H) from her mother, she is at risk of
developing Huntington’s disease. If she inherits the normal gene (h) from
both parents, then she would not develop the disease. However, we would
need to know her specific genotype (Hh or hh), to be able to determine
the exact probability.
23.
Autosomal Recessive Inheritance (trait only expressed if
homozygous recessive)
Cystic fibrosis (CF) is a recessive genetic disease that leads to persistent
lung infections reducing the ability to breathe over time.
Patients with CF
have a mutation in the CFTR gene which prevents the movement of
chloride – a component of salt – across cell membranes. This makes
mucus in the body very thick and sticky so that it clogs airways and traps
bacteria. It also prevents digestive enzymes from releasing and causes
poor nutrition. CF is very prevalent in Caucasians of northern European
ancestry. A
carrier
is a person who appears normal but carries the
recessive allele for a disease. If two parents are both carriers
(Cc
)
for CF,
what is the chance they will have a child with CF?
25% or 1 in 4 chances
The chances are: CC (normal), Cc (carrier), cc (affected with cystic
fibrosis)
24.
Von Willebrand Disease is an autosomal dominant disorder (not located
on the sex chromosomes) where blood will not clot properly. What would
be the two possible genotypes of a person who has the disorder?
(VV)
,
(Vv)
25.
If a person is heterozygous for the trait (having the disease) is married
to a normal spouse (dd), what is the chance that their children will have
the disorder?
50%
X-Linked Disorders:
Recall that in many organisms, the determination of sex involves a pair
of chromosomes that differ in length and genetic content. These
chromosomes are called the sex chromosomes. Different organisms have
different systems. For today we will focus on the human system where males
are XY and females are XX. The X chromosome carries hundreds of genes
which are not necessary for sex determination but are necessary for correct
development and viability of the offspring. These genes are not present on
the Y chromosome and so display the characteristic pattern of inheritance
called sex-linkage or more commonly X-linkage.
Any XY individual that inherits an
X-linked recessive
disease allele
will be affected by it, because they do not have a second copy of the X
chromosome to provide a dominant allele. Therefore, in X-linked recessive
inheritance, XY individuals, commonly males, tend to be affected more
frequently than XX individuals, commonly females, in a population (see
Figure 8).
Figure 8:
Some forms of color blindness are inherited as X-linked recessive traits.
Color blindness is diagnosed using tests such as this. Ishihara Test. (Wikipedia-
unknown-PD) (right) A pedigree consistent with XR inheritance. (Original-Deyholos-
CC:AN)
The reverse is true for
X-linked dominant
inheritance, where the
gene responsible for the disease is located on the X-chromosome, and the
allele that causes the disease is dominant. In this case XX individuals, tend
to be more frequently affected than XY individuals in the population because
they have two copies of the X chromosome (see Figure 9).
Your preview ends here
Eager to read complete document? Join bartleby learn and gain access to the full version
- Access to all documents
- Unlimited textbook solutions
- 24/7 expert homework help
Figure 9:
Some types of rickets may follow an XD mode of inheritance. (Wikipedia-
Mrish-CC:AS) (middle and left) Two pedigrees consistent with XD inheritance.
(Original-Deyholos_CC:AN)
Keep in mind that in autosomal dominant and recessive traits, the sex
of the individual does not change the probability of being affected, because
all individuals have two copies of autosomes.
Questions:
26.
In humans, hemophilia is a sex-linked trait. Females can be normal,
carriers, or have the disease. Males will either have the disease or not
(but they won’t ever be carriers).
X
H
X
H
= female, non-hemophilic
X
H
Y = male, non-hemophilic
X
H
X
h
= female, carrier
X
h
X
h
= female, hemophiliac
X
h
Y= male, hemophiliac
Show the cross of a man who has hemophilia with a woman who is a
carrier.
What is the probability that their children will have the disease?
2 out
of 4, 50%
27.
A woman who is a carrier marries a non-hemophilic man. Show the
cross. What is the probability that their children will have hemophilia?
50%
What sex will a child in the family with hemophilia be?
Male (XhY),
hemophilia sex linked trait
28.
A woman who has hemophilia marries a non-hemophilic man. How
many of their children will have hemophilia, and what is their sex?
50% of their children. Male (XhY) and female (XhXh)
possible genotypes:
XHY (male non-hemophilic), XhY (male, hemophilic)
XhXH (female, carrier), XhXh (female, hemophilic)
29.
The possible genotypes and phenotypes for an x-linked recessive
disorder color-blindness is as follows:
X
B
X
B
= female, normal vision
X
B
X
b
= female, carrier
X
b
X
b
= female, color blindness
X
B
Y = male, normal vision
X
b
Y= male, color blindness
Show the cross of a female carrier with a normal vision male.
What is the probability that their children will have color blindness?
50%
What gender will they be?
Both male and fermale
30.
A woman with normal vision, whose father was color blind, marries a
man with normal vision. What genotypes are possible in their offspring?
Female normal vision X
B
X, Male normal vision X
B
Y, Female carrier X
b
X,
Male color blindness X
b
Y
31.
Calico is a coat color found in cats, which is caused by a SEX-LINKED,
CODOMINANT allele.
Female cats can be black X
B
X
B
, orange X
R
X
R
, or calico X
B
X
R
Male cats can be black X
B
Y or orange X
R
Y
A black male is crossed with an orange female
How many kittens are: orange males?
2
calico females?
2
32.
An orange male is crossed with a calico female. Show the Punnett
square and list the phenotypes and proportions.
Phenotype= 3:1
33.
A black male is crossed with a black female. Show the Punnett square
and list the phenotypes and proportions.
Phenotype= 2:1
Your preview ends here
Eager to read complete document? Join bartleby learn and gain access to the full version
- Access to all documents
- Unlimited textbook solutions
- 24/7 expert homework help
34.
A black male is crossed with a calico female. Show the Punnett square
and list the phenotypes and proportions.
Phenotype= 3:1
Part 4: Human Traits not Governed by Mendelian Genetics
- Multiple Alleles and Codominance
There are many exceptions to Mendel’s Rules. For example, blood types in
humans exhibit two exceptions: codominance and multiple alleles.
When a trait is controlled by
multiple alleles
, the gene will have more
than two possible alleles. However, each individual can still only ever have
two alleles, one from each chromosome given by their parents. The classic
example of multiple alleles is human blood types – A, B and O. The alleles are
denoted as:
I
A
, I
B
, i
. We do not use O to denote the allele, as O and o are too
similar and mistaking blood types is almost always fatal.
Red blood cells present antigens on their surface which act as passes
allowing the red blood cells to move through the body without being
mistaken for an intruders or pathogens. Individuals with the
I
A
allele will have
the A antigen present on their red blood cells. The same for the
I
B
allele,
which causes the B antigen to be presented. If a person has both the
I
A
and
I
B
alleles their red blood cells will present both A and B antigens, this is called
codominance
. Conversely, if an individual has the
i
allele their red blood
cells will not present any antigen. See Figure 10 below.
Figure 10:
Representation of red blood cells and the antigens they carry. " Human
Inheritance" by LibreTexts is licensed under CC BY-NC-SA 3.0.
Table 4: Basic Genotypes, Antigens and Blood types of Humans
Genotypes
Antigens on Blood
Cells
Blood Type
I
A
i , I
A
I
A
A
A
I
B
i, I
B
I
B
B
B
I
A
I
B
A and B
AB
ii
None
O
Questions:
35.
If two individuals with blood type AB marry, what are the odds that
each of their children will have the AB blood type?
IAIAType A: 25%; IAIB Type B: 25%; IBIB Type AB: 50%
36.
A disputed paternity case! Hermione’s new baby has a blood type of O.
Her blood type is B and Ron Weasley’s is A. Harry Potter (blood type AB)
insists he is the child’s father. CAN THIS BE TRUE?
No, it is not true
because Hermione Type B (IBIB or IBi) and Ron Type A (IAIA or IAi) could
both pass O alleles to their child, resulting in a type O (ii) blood child. It is
impossible for Harry blood type AB (IAIB) to be the father.
37.
A woman and her son are both blood type O. The woman claims that a
man with blood type A is the father of the boy. Is this possible? Explain
your answer.
Yes, only when the father is heterozygous A or B or if the
father has O blood group can this be possible.
38.
In a recent case in Spokane, Washington, a woman claimed a man was
the father of her child. The man denied it. The man’s lawyer demanded
that blood types be taken to prove the innocence of his client. The
following results were obtained: Alleged father, Type O. Mother, Type A.
Child, Type AB.
a.
What are the possible genotypes for these three people?
Alleged
father(O): (ii) Mother (A): IAIA or IAi , Child (AB): IAIB
b.
Do you agree with the court’s decision? Why or why not?
Don’t
agree. The father’s blood group is O (ii) and therefore cannot
contribute the B allele to the child. The man and woman would
produce O or A; or O or A, O.
39.
It was suspected that two babies had been exchanged in a hospital. Mr.
and Mrs. Jones received baby #1 and Mr. and Mrs. Simon received baby
#2. Blood typing tests on the parents and the babies showed the
following:
1.
Mr. Jones: Type A
(IAIA or IAi)
2.
Mrs. Jones: Type O
(ii)
3.
Baby #1: Type A
(IAi)
4.
Mr. Simon: Type AB
(IAIB)
5.
Mrs. Simons: Type O
(ii)
6.
Baby #2 Type O
(ii)
Were the babies switched? Do you know this for sure?
Yes. because Mr.
Simons cannot produce O offspring; therefore, baby 2 belongs to the
Jones, and baby 1 belongs to the Simons.
Part 5: Identification of Chromosomes and Chromosomal
Abnormalities Using Karyotypes
Although Mendel is referred to as the “father of modern genetics,” he
performed his experiments with none of the tools that the geneticists of
today routinely employ
. One such powerful cytological technique is
karyotyping
, a method in which traits characterized by chromosomal
abnormalities can be identified from a single cell. To observe an individual’s
karyotype, a person’s cells (like white blood cells) are first collected from a
Your preview ends here
Eager to read complete document? Join bartleby learn and gain access to the full version
- Access to all documents
- Unlimited textbook solutions
- 24/7 expert homework help
blood sample or other tissue. In the laboratory, the isolated cells are
stimulated to begin actively dividing. A chemical called colchicine is then
applied to cells to arrest condensed chromosomes in metaphase. Cells are
then made to swell using a hypotonic solution, so the chromosomes spread
apart. Finally, the sample is preserved in a fixative and applied to a slide.
The geneticist then stains chromosomes with one of several dyes to
better visualize the distinct and reproducible banding patterns of each
chromosome pair. Following staining, the chromosomes are viewed using
bright-field microscopy.
A common stain choice is the Giemsa stain. Giemsa
staining results in approximately 400–800 bands (of tightly coiled DNA and
condensed proteins) arranged along all of the 23 chromosome pairs. An
experienced geneticist can identify each chromosome based on its
characteristic banding pattern. In addition to the banding patterns,
chromosomes are further identified on the basis of size and centromere
location. To obtain the classic depiction of the karyotype in which
homologous pairs of chromosomes are aligned in numerical order from
longest to shortest, the geneticist obtains a digital image, identifies each
chromosome, and manually arranges the chromosomes into this pattern.
At its most basic, the karyotype may reveal genetic abnormalities in which
an individual has too many or too few chromosomes per cell. Examples of
this are
Down Syndrome
, which is identified by a third copy of chromosome
21, and
Turner Syndrome
, which is characterized by the presence of only
one X chromosome in women instead of the normal two.
Geneticists can also
identify large deletions or insertions of DNA.
For instance,
Jacobsen
Syndrome
, which involves distinctive facial features as well as heart and
bleeding defects, is identified by a deletion on chromosome 11.
Finally, the
karyotype can pinpoint translocations, which occur when a segment of
genetic material breaks from one chromosome and reattaches to another
chromosome or to a different part of the same chromosome. Translocations
are implicated in certain cancers, including chronic myelogenous leukemia.
Your preview ends here
Eager to read complete document? Join bartleby learn and gain access to the full version
- Access to all documents
- Unlimited textbook solutions
- 24/7 expert homework help
Figure 11:
A human karyotype: This karyotype is of a male human. Notice that
homologous chromosomes are the same size and have the same centromere
positions and banding patterns. A human female would have an XX chromosome
pair instead of the XY pair shown.
Key Points:
A karyotype depicts the number, size, and any abnormalities of the
chromosomes in an organism.
A normal human karyotype contains 23 pairs of chromosomes: 22 pairs
of autosomes and 1 pair of sex chromosomes, generally arranged in
order from largest to smallest.
The
short arm of a chromosome is
referred to as the p arm, while the
long arm is
designated the q arm.
A karyotype can be used to visualize abnormalities in the
chromosomes, such as an incorrect number of chromosomes,
deletions, insertions, or translocations of DNA.
Your preview ends here
Eager to read complete document? Join bartleby learn and gain access to the full version
- Access to all documents
- Unlimited textbook solutions
- 24/7 expert homework help
Questions:
Analyze this karyotype and determine if there is an abnormality with the sex
chromosomes.
40.
What is the sex chromosome makeup?
The sex chromosome makeup is
XYY. A male receives an extra Y chromosome from his father.
41.
Do a search of your results and determine what syndrome the patient
had. Write it and some of its symptoms here.
It is the Jacobsen Syndrome,
aka 47,XYY syndrome. Boys might be taller than other boys, they will also
possess problems with spoken language and processing spoken words,
coordination problems, weaker muscles, hand tremors and behavioral
problems.
42.
Do a search of your results and determine what syndrome the patient
had. Write it and some of its symptoms here.
It is Turner syndrome,
genetic makeup of (45, X0). Symptoms include short stature, delayed
puberty, infertility, heart defects, and certain learning disabilities.
Analyze this karyotype and determine if there is an abnormality with the sex
chromosomes.
43.
What is the sex chromosome makeup?
The sex chromosome makeup
is 45,X, or 45,XO
Your preview ends here
Eager to read complete document? Join bartleby learn and gain access to the full version
- Access to all documents
- Unlimited textbook solutions
- 24/7 expert homework help
44.
What syndrome does this individual exhibit?
b. Turner Syndrome
a.
Klinefelter syndrome
b.
Turner Syndrome
c.
Poly-X syndrome
d.
Jacob syndrome
Your preview ends here
Eager to read complete document? Join bartleby learn and gain access to the full version
- Access to all documents
- Unlimited textbook solutions
- 24/7 expert homework help
Part 6: Pedigree Analysis
Geneticists illustrate the inheritance of a gene within a family by using
a pedigree chart. In a pedigree chart, males are symbolized by a square (□)
and females are symbolized by a circle (o). People who are
affected by a
condition or disease are symbolized by a dark/filled square or circle
.
Carriers
are
often symbolized
by a half filled square or circle.
The pedigree chart below shows the inheritance of albinism in three
generations of a family. The couple labeled 1 and 2 had five children,
including one albino daughter (5). One of the sons (3) and his wife (4) had
four children, including one albino son (6).
Questions:
45.
You will write the genotypes of each individual who is labeled with a
number in the pedigree. Use 'A' to represent the dominant allele and 'a' to
represent the recessive allele. Begin by writing in the genotypes of 5 and
6. How do you know their genotypes?
1=Aa, 2=Aa, 3=Aa, 4=Aa
Genotype of 5: aa
and Genotype of 6: aa
With parents being heterozygous with the condition, they are carriers;
therefore, the offspring will have defective/mutated genes.
46.
Explain how you can determine the genotypes of 1 and 2. Include
Punnett Square for these parents in your explanation. Write their
genotypes in the pedigree.
Genotype of 1: Aa
and Genotype 2: Aa
To determine the genotype of 1
and 2 being that they are both heterozygous, meaning both have a
dominant allele and a recessive allele, which the recessive allele is then
inherited by two of the offspring.
Your preview ends here
Eager to read complete document? Join bartleby learn and gain access to the full version
- Access to all documents
- Unlimited textbook solutions
- 24/7 expert homework help
47.
Write the genotypes of 3 and 4 in the pedigree.
Genotype of 3: Aa
Genotype of 4: Aa
48.
Explain how you can figure out the genotype of 7 and write her
genotype in the pedigree.
So, 5 (aa) suffers from albinism, it is
homozygous for recessive gene, but the offspring from 5 (aa) do not suffer
from albinism, this means that the male is a homozygous dominant gene
and the genotype of 7: Aa
Many other conditions are the result of homozygous recessive alleles, so
these conditions are inherited in the same manner as albinism. These
include:
Cystic fibrosis (a genetic disease that results in difficulty in breathing
and serious illness)
Phenylketonuria (a genetic disease that results in mental retardation
unless phenylketonuria is detected at birth and treated with a special
diet).
This pedigree shows the inheritance of a different condition called
achondroplasia (ay-kon-druh-play-zhuh), a form of dwarfism. Dark circles or
squares represent individuals with achondroplasia.
49.
Think about 5 and 6 and their children. Based on this family, is the
allele that causes
achondroplasia recessive or dominant? How do you
know? Include a Punnett square for 5 and 6 and their children in your
answer. Use 'D' to represent the dominant allele and 'd' to represent the
recessive allele.
The allele is dominant because the presence of just one D
allele in the genotype results in the trait. In the Punnett Square, there are
Your preview ends here
Eager to read complete document? Join bartleby learn and gain access to the full version
- Access to all documents
- Unlimited textbook solutions
- 24/7 expert homework help
3 combination that result in achondroplasia: DD, Dd, Dd and only one
combination that does not which is dd.
50.
Write the genotypes of 5 and 6 in the pedigree.
5=Dd, 6=Dd
51.
Write the genotypes of 2, 3 and 7 in the pedigree. How do you know
their genotypes?
2=dd, 3=dd, 7=dd
These are homozygous for the
recessive allele (dd) as they have unaffected offspring.
52.
Determine the genotypes of 1 and 4. Show Punnett Square and
explain your reasoning. Write the genotypes of 1 and 4 in the pedigree.
1=Dd, 4=Dd
53.
Most people who have the achondroplasia allele did not inherit this
allele from their parents. For a person who has the achondroplasia allele,
but did not inherit it from his or her parents, what biological process is the
most likely explanation for this person’s achondroplasia allele?
The
biological process likely to explain achondroplasia allele in a person who
has the achondroplasia allele but wasn’t inherited by their parents is
known as de novo mutation which is a new mutation that occurs in a
person’s DNA which isn’t present in either parent. It can happen during
the formation of reproductive cells of the sperm/egg or early in embryonic
development.
Licenses and Attributions:
" Human Inheritance"
by
LibreTexts
is licensed under
notset
.
" A Taste of Genetics - PTC Taster"
by
Orange County Biotechnology
Education Collaborative
,
LibreTexts
is licensed under
CC BY-NC-SA
.
T
itle taken from the lab developed by
Embi Tec
and used with
permission.
Your preview ends here
Eager to read complete document? Join bartleby learn and gain access to the full version
- Access to all documents
- Unlimited textbook solutions
- 24/7 expert homework help
Biologycorner by
Shannan Muskopf
is licensed under a
Creative
Commons Attribution-NonCommercial-ShareAlike 4.0 International
License
.
" Inferring the Mode of Inheritance"
by
Todd Nickle and Isabelle
Barrette-Ng
,
LibreTexts
is licensed under
CC BY-SA
.
" Identification of Chromosomes and Karyotypes"
by
LibreTexts
is
licensed under
CC BY-NC-SA 3.0
.
By Dimitrios Athanatos, Christos Tsakalidis, George P Tampakoudis,
Maria N Papastergiou, Fillipos Tzevelekis, George Pados, and Efstratios
A Assimakopoulos -
https://www.ncbi.nlm.nih.gov/pmc/articles/PMC2769437/, CC BY 3.0
https://commons.wikimedia.org/w/index.php?curid=107263520
https://commons.wikimedia.org/w/index.php?curid=1285283
, CC BY-
SA 3.0
" Genetics Supplementary Document"
by
LibreTexts
is licensed
under
CC BY-NC
.
Your preview ends here
Eager to read complete document? Join bartleby learn and gain access to the full version
- Access to all documents
- Unlimited textbook solutions
- 24/7 expert homework help
Related Documents
Recommended textbooks for you
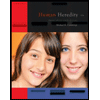
Human Heredity: Principles and Issues (MindTap Co...
Biology
ISBN:9781305251052
Author:Michael Cummings
Publisher:Cengage Learning
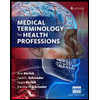
Medical Terminology for Health Professions, Spira...
Health & Nutrition
ISBN:9781305634350
Author:Ann Ehrlich, Carol L. Schroeder, Laura Ehrlich, Katrina A. Schroeder
Publisher:Cengage Learning
Recommended textbooks for you
- Human Heredity: Principles and Issues (MindTap Co...BiologyISBN:9781305251052Author:Michael CummingsPublisher:Cengage Learning
- Medical Terminology for Health Professions, Spira...Health & NutritionISBN:9781305634350Author:Ann Ehrlich, Carol L. Schroeder, Laura Ehrlich, Katrina A. SchroederPublisher:Cengage Learning
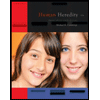
Human Heredity: Principles and Issues (MindTap Co...
Biology
ISBN:9781305251052
Author:Michael Cummings
Publisher:Cengage Learning
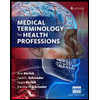
Medical Terminology for Health Professions, Spira...
Health & Nutrition
ISBN:9781305634350
Author:Ann Ehrlich, Carol L. Schroeder, Laura Ehrlich, Katrina A. Schroeder
Publisher:Cengage Learning